In the past few decades, animal rights issues have been an emerging topic, with debate growing louder, especially relating to the suffering that accompanies raising animals for human food production. THINK talks to Australian philosopher Peter Singer to discuss animal ethics.
Continue readingWitnessing the Hong Kong Student Protests
THINK revisits the cause and effect of a political movement that was led by students and felt across the globe.
Continue readingProtecting the World’s largest experiment
The particle beams circulating in the CERN Large Hadron Collider (LHC) have enough energy to melt 500kg of copper. How can we protect the machine from itself?
My phone rang, waking me up in the middle of the night. It is 2 a.m., and I (Dr Gianluca Valentino) am driving from the sleepy French village where I live at the foot of the snow-capped Jura mountains to the CERN Control Centre. As groggy as I feel, I am trembling with excitement at finally putting months of my work to the test.
The operators on night shift greet me as I come in through the sliding door. These are the men and women who keep the €8 billion Large Hadron Collider (LHC) running smoothly. The LHC produces 600 million particle collisions per second to allow physicists to examine the fundamentals of the universe. Their most recent discovery is the Higgs boson, a fundamental particle. In 2012, this finding appears to have confirmed the Higgs field theory, which describes how other particles have mass. It helps explain the universe around us.
The empty bottles of champagne on the shelves of the CERN Control Centre are a testimony to the work of thousands of physicists, engineers, and computer scientists. The LHC has now busted record after record rising to stratospheric fame.
The LHC is an engineering marvel. A huge circular tunnel 100 m underground and 27 km in circumference. It straddles the Franco-Swiss border and is testimony to the benefit of 50 years of non-military research at CERN, the European Organization for Nuclear Research. CERN is also the birthplace of the World Wide Web.
The LHC works by colliding particles together. In this way physicists can peer into the inner workings of atoms. Two counter-rotating hadron (proton or heavy-ion) beams are accelerated to approach the speed of light using a combination of magnetic and electric fields. A hadron is a particle smaller than atoms, and is made up of several types of quarks, which are fundamental particles (there is nothing smaller than them — for now). The beams circulate at an energy of 7 TeV, which is similar to a French TGV train travelling at 150 km per hour.
For the magnets to work at maximum strength, they need to operate in super-conducting mode. This mode needs the collider to be cooled to -271°C using liquid helium, making it the coldest place in the universe. It is also the hottest place in the universe. Collisions between lead ions have reached temperatures of over 5 trillion °C. Not even supernovae pack this punch.
The two separate beams are brought together and collided at four points where the physics detectors are located. A detector works by gathering all the information generated by the collisions which generate new sub-atomic particles. The detectors track their speed and measure the energy and charge. ‘Gluon fusion’ — when two gluons combine (a type of boson or particle that carries a force) — is the most likely mechanism for Higgs boson production at the LHC.
My role in this huge experiment is to calibrate the LHC’s brakes. Consider a simple analogy. A bike’s brakes need to be positioned at
the right distance from the circulating wheel, and are designed to halt a bike in its tracks from a speed of around 70 km/hr. Too far apart, and when the brakes are applied, the bike won’t stop. Too close together, and the bike won’t even move. In the LHC, the particles travel at nearly 300,000 km/s.
Equipment called collimators act as the LHC’s brakes. The LHC is equipped with 86 of them, 43 per beam. They passively intercept particles travelling at the speed of light, which over time drift from the centre outwards. The machine is unprotected if the collimators are placed too far away from the beam. The beam’s energy, equivalent of 80 kg of TNT, would eventually drill a hole needing months or years to repair. If the collimators are too close to the beam they sweep up too many particles, reducing the beam’s particle population and its lifetime.
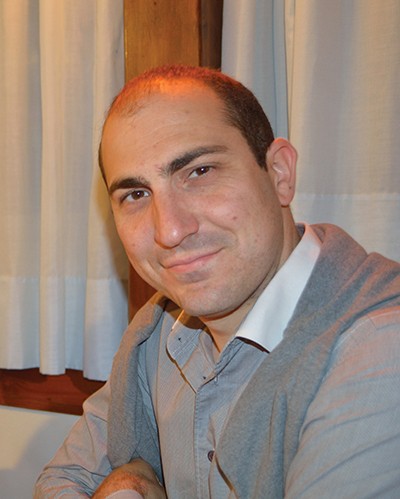
The LHC has four different types of collimators, which clean the particles over multiple stages in the space of a few hundred metres. These collimators also protect the expensive physics detectors from damage if a beam were to hit them directly. If the detectors were hit the LHC would grind to a halt.
What does it take to calibrate these brakes? Each brake or collimator is made up of two metre-long blocks of carbon composite or tungsten, known as ‘jaws’. The jaws should be positioned symmetrically on either side of the beam, and opened to gaps as small as 3 mm to let the beam through. They can be moved in 5 µm increments— that is 20 times less than the width of a typical human hair. The precision is necessary but makes the procedure very tedious.
The beam’s position and size at each collimator are initially unknown. They are determined through a process called beam-based alignment. During alignment, each jaw is moved in steps towards the beam, until it just scrapes the edge. Equipment near the collimator registers the amount of particles they are mopping up. Then, the beam position is calculated as the average of the aligned jaw positions on either side, while the beam size is determined from the jaw gap.
The problem is that there are 86 collimators. Each one needs to be calibrated making the process painfully slow. To calibrate the jaws manually takes several days, totaling 30 hours of beam time. To top it all, the alignment has to be repeated at various stages of the machine cycle, as the beams shrink with increasing energy, and imperfections in the magnetic fields can lead to changes in the beam’s path. This wasted time costs millions and makes the LHC run slower.
Previously, one had to click using a software application for each jaw movement towards the beam. With a step size of 5 µm and a total potential distance of 10 mm, that is 2000 clicks per collimator jaw! Extreme precision is required when moving the collimator jaws. If the jaw moves too much into the beam, the particle loss rate will exceed a certain threshold, and the beam is automatically extracted from the LHC. A few hours are wasted until the operators get the machine back and the alignment procedure is restarted.
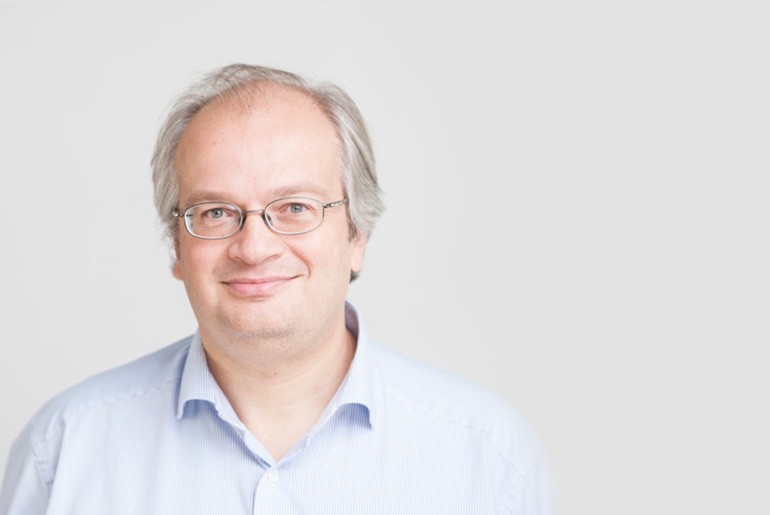
Over the course of my Ph.D., I automated the alignment, speeding it up by developing several algorithms — computer programs that carry out a specific task. The LHC now runs on a feedback loop that automatically moves the jaws into the correct place without scraping away too much beam. The feedback loop enables many collimators to be moved simultaneously, instead of one at a time. A pattern recognition algorithm determines whether the characteristic signal observed when a jaw touches the beam is present or not. This automates what was previously a manual, visual check performed by the operator.
The sun’s rays begin to filter through the CERN Control Centre, and the Jura mountains are resplendent in their morning glory. The procedure is complete: all collimators are aligned in just under 4 hours, the fastest time ever achieved.
In early 2013, the LHC was shut down for a couple of years for important upgrades. Before then my algorithms helped save hundreds of hours; since the LHC costs €150,000 per hour to run, millions of euros were also saved. This software was part of the puzzle to provide more time for the LHC’s physics programme and is now here to stay.
The morning shift crew comes in. The change of guard is performed to keep the machine running 24 hours a day, 7 days a week, while I head home to catch up on lost sleep.
What is a collimator?
How does a particle accelerator work?
Find Out More
INDIE GAMES
How can a video game ask questions about life, art, and frustration? Giuliana Barbaro-Sant met up with Dr Pippin Barr to tell us about his game adaptation of Marina Abramović‘s artwork The Artist is Present.
In each creative act, a personal price is paid. When the project you have been working on so hard falls to pieces because of funding, it is hard to accept its demise. The feeling of failure, betrayal, and loneliness is an easy trap to fall into. This is the independent game maker’s industry: a bloodthirsty world rife with competition, sucking pockets dry from the very beginning of the creative process.
Maltese game makers face a harsher reality. Not all game makers are lucky enough to make it to the finish line, publish, and make good money. Rather, most of them rarely do. Yet, if and when they get there, it is often thanks to the passion and dedication they put into their creation — together with the continuous support of others.
Dr Pippin Barr always had a passion for making things, be it playing with blocks or doodling. His time lecturing at the Center for Computer Game Research at the IT University of Copenhagen, together with his recent team-up with the newly opened Institute of Digital Games at the University of Malta, only served to reincarnate another form of this passion: Pippin makes games. At the Museum of Modern Art in New York he exhibited his most well known work: the game rendition of Marina Abramović’s The Artist is Present. He thought of the idea while planning to deliver lectures about how artists invoke emotions through laborious means in their artworks. In The Artist is Present, artist Marina Abramović sits still in front of hundreds of thousands of people and just stares into their eyes for as long as participants desire.
There is more to this performance than meets the eye. Beyond the simplistic façade, Barr saw real depth. Through eye contact, the artist and audience forge a unique connection. All barriers drop, and human emotion flows with a great rawness that games are so ‘awful’ at embodying. Yet, paradoxically, there is a militariness in the preparation behind the performance that games embrace only too well. Not only does the artist have to physically programme herself to withstand over 700 hours’ worth of performing, but the audience also prepares for the experience in their own way, by disciplining themselves as they patiently wait for their turn.
“It’s a pretty lonely road and it can be tough when you’re stuck with yourself”
Pippin Barr
‘Good research is, after all, creative,’ according to Pippin Barr. By combining his academic background with his creative impulse, he made an art game — a marriage between art and video games. These are games about games, which test their values and limits. Barr relishes the very idea of questioning the way things work. His self-reflexive games serve as a platform for him to call into question life’s so-called certainties, in a way that is powerful enough to strike a chord in both himself and the player. He is looking to create a deep emotional resonance, which gives the player a chance to ‘get’ the game through a unique personal experience. Sometimes, players write about his games and capture what Pippin Barr was thinking about, as he put it, ‘better than I could myself’, or read deeper than his own thoughts.
As far as gameplay goes, The Artist is Present is fairly easy to manoeuvre in. The look is fully pixellated yet captures the ambience at the Museum. The first screen of the game places the player in front of its doors and you are only allowed in if you are playing the game during the actual exhibition’s opening hours in America. Until then, there is no option but to wait till around 4:30 pm our time (GMT+1). The frustration continues increasing since after entering you will still have to wait behind a long queue of strangers to experience the performance work. This reflects real world participants who had to wait to experience The Artist is Present. If they were lucky, they sat in front of the artist and gazed at her for as long as they wanted.
Interestingly, Marina Abramović also played the game. She told Barr about how she was kicked out of the queue when she tried to catch a quick lunch in the real world as she was queuing in the digital one. Very unlucky, but the trick is to keep the game tab open. Other than that, good luck!
Despite that little hiccup, Abramović did not give up on the concept of digitalising the experience of her art. After The Artist is Present, Barr and Abramović set forth on a new quest: the making of the Digital Marina Abramović Institute. Released last October, it has proven to be a great challenge for those who cannot help but switch windows to check up on their Facebook notifications – not only are the instructions in a scrolling marquee, but you have to keep pressing the Shift button on your keyboard to prove you are awake and aware of what is happening in the game. It is the same kind of awareness that is expected out of the physical experience of the real-life Institute.
The quirkiness of Barr’s games reflects their creator. Besides The Artist is Present, in Let’s Play: Ancient Greek Punishment, he adapted a Greek Sisyphus myth to experiment with the frustration of not being rewarded. In Mumble Indie Bungle, he toyed with the cultural background of indie game bundles by creating ‘terrible’ versions with ‘misheard titles’ (and so, ‘misheard’ game concepts) of renowned indie games. One of his 2013 projects involves the creation of an iPhone game, called Snek, an adaptation of the good old Nokia 3310 Snake. In his version, Pippin Barr turned the effect of the smooth ‘naturally’ perfect touch interface of the device upon its head, by using the gyroscope feature. Instead, the interaction with the Apple device becomes thoroughly awkward, as the player has to move around very unnaturally because of the requirements of the game.
This dedicated passion for challenging boundaries ultimately drives creators and artists alike to step out of their comfort zone and make things. These things challenge the way society thinks and its value systems. Game making is no exception, especially for independent developers. An artist yearns for the satisfaction that comes with following a creative impulse and succeeding. In Barr’s case, being ‘part of the movement to expand game boundaries and show players (and ourselves) that the possibilities for what might be “allowed” in games is extremely broad.’
Accomplishing so much, against the culture industry’s odds, is a great triumph for most indie developers. For Pippin Barr, the real moment of success is when the game is finished and is being played. Then he knows that someone sat with the game and actually had an experience — maybe even ‘got it’.
Follow Pippin Barr on Twitter: @pippinbarr or on: www.pippinbarr.com
Giuliana Barbaro-Sant is part of the Department of English Master of Arts programme.
An Intelligent Pill
Doctors regularly need to use endoscopes to take a peek inside patients and see what is wrong. Their current tools are pretty uncomfortable. Biomedical engineer Ing. Carl Azzopardi writes about a new technology that would involve just swallowing a capsule.
Michael* lay anxiously in his bed, looking up at his hospital room ceiling. ‘Any minute now’, he thought, as he nervously awaited his parents and doctor to return. Michael had been suffering from abdominal pain and cramps for quite some time. The doctors could not figure it out through simple examinations. He could not take it any more. His parents had taken him to a gut specialist, a gastroenterologist, who after asking a few questions, had simply suggested an ‘endoscopy’ to examine what is wrong. Being new to this, Michael had immediately gone home to look it up. The search results did not thrill him.
The word ‘endoscope’ derives from the Greek words ‘endo’, inside, and ‘scope’, to view. Simply put, looking inside our body using instruments called endoscopes. In 1804, Phillip Bozzini created the first such device. The Lichtleiter, or light conductor, used hollow tubes to reflect light from a candle (or sunlight) onto bodily openings — rudimentary.
Modern endoscopes are light years ahead. Constructed out of sleek, black polyurethane elastometers, they are made up of a flexible ‘tube’ with a camera at the tip. The tubes are flexible to let them wind through our internal piping, optical fibers shine light inside our bodies, and since the instrument is hollow it allows forceps or other instruments to work during the procedure. Two of the more common types of flexible endoscopes used nowadays are called gastroscopes and colonoscopes. These are used to examine your stomach and colon. As expected, they are inserted through your mouth or rectum.
Michael was not comforted by such advancements. He was not enticed by the idea of having a flexible tube passed through his mouth or colon. The door suddenly opened. Michael jerked his head towards the entrance to see his smiling parents enter. Accompanying them was his doctor holding a small capsule. As he handed it over to Michael, he explained what he was about to give him.
Enter capsule endoscopy. Invented in 2000 by an Israeli company, the procedure is simple. The patient just needs to swallow a small capsule. That is it. The patient can go home, the capsule does all the work automatically.
The capsule is equipped with a miniature camera, a battery, and some LEDs. It starts to travel through the patient’s gut. While on its journey it snaps around four to thirty-five images every second. Then it transmits these wirelessly to a receiver strapped around the patient’s waist. Eventually the patient passes out the capsule and on his or her next visit to the hospital, the doctor can download all the images saved on the receiver.
The capsule sounds like simplicity itself. No black tubes going down patients’ internal organs, no anxiety. Unfortunately, the capsule is not perfect.
“The patient just needs to swallow a small capsule. That is it. The patient can go home, the capsule does all the work automatically”
First of all, capsule endoscopy cannot replace flexible endoscopes. The doctors can only use the capsules to diagnose a patient. They can see the pictures and figure out what is wrong, but the capsule has no forceps that allow samples to be taken for analysis in a lab. Flexible endoscopes can also have cauterising probes passed through their hollow channels, which can use heat to burn off dangerous growths. The capsule has no such means. The above features make gastroscopies and colonoscopies the ‘gold standard’ for examining the gut. One glaring limitation remains: flexible endoscopes cannot reach the small intestine, which lies squarely in the middle between the stomach and colon. Capsule endoscopy can examine this part of the digestive tract.
A second issue with capsules is that they cannot be driven around. Capsules have no motors. They tend to go along for the ride with your own bodily movements. The capsule could be pointing in the wrong direction and miss a cancerous growth. So, the next generation of capsules are equipped with two cameras. This minimises the problem but does not solve it completely.
The physical size of the pill makes these limitations hard to overcome. Engineers are finding it tricky to include mechanisms for sampling, treatment, or motion control. On the other hand, solutions to a third problem do exist. This difficulty relates to too much information. The capsule captures around 432,000 images over the 8 hours it snaps away. The doctor then needs to go through nearly all of these images to spot the problematic few. A daunting task that uses up a lot of time, increasing costs, and makes it easier to miss signs of disease.
A smart solution lies in looking at image content. Not all images are useful. A large majority are snapshots of the stomach uselessly churning away, or else of the colon, far down from the site of interest. Doctors usually use capsule endoscopy to check out the small intestine. Medical imaging techniques come in handy at this point to distinguish between the different organs. Over the last year, the Centre for Biomedical Cybernetics (University of Malta) has carried out collaborative research with Cardiff University and Saint James Hospital to develop software which gives doctors just what they need.
Following some discussions between these clinicians and engineers they quickly realised that images of the stomach and large intestine were mostly useless for capsule endoscopes.
Identifying the boundaries of the small intestines and extracting just these images would simplify and speed up screening. The doctor would just look at these images, discarding the rest.
Engineers Carl Azzopardi, Kenneth Camilleri, and Yulia Hicks developed a computer algorithm that could first and foremost tell the difference between digestive organs. An algorithm is a bit of code that performs a specific task, like calculating employees’ paychecks. In this case, the custom program developed uses image-processing techniques to examine certain features of each image, such as colour and texture, and then uses these to determine which organ the capsule is in.
Take colours for instance. The stomach has a largely pinkish hue, the small intestine leans towards yellowish tones, while the colon (unsurprisingly perhaps) changes into a murky green. Such differences can be used to classify the different organs. Additionally, to quickly sort through thousands of images, the images need to be compacted. A specific histogram is used to amplify differences in colour and compress the information. These procedures make it easier and quicker for algorithm image processing.
Texture is another unique organ quality. The small intestine is covered with small finger-like projections called villi. The projections increase the surface area of the organ, improving nutrient absorption into the blood stream. These villi give a particular ‘velvet-like’ texture to the images, and this texture can be singled out using a technique called Local Binary Patterns. This works by comparing each pixel’s intensity to its neighbours’, to determine whether these are larger or smaller in value than its own. For each pixel, a final number is then worked out which gauges whether an edge is present or not (see image).
Classification is the last and most important step in the whole process. At this point the software needs to decide if an image is part of the stomach, small intestine, or large intestine. To help automatically identify images, the program is trained to link the factors described above with the different organ types by being shown a small subset of images. This data is known as the training set. Once trained, the software can then automatically classify new images from different patients on its own. The software developed by the biomedical engineers was tested first by classification based just on colours or texture, then testing both features together. Factoring both in gave the best results.
“The software is still at the research stage. That research needs to be turned into a software package for a hospital’s day-to-day examinations”
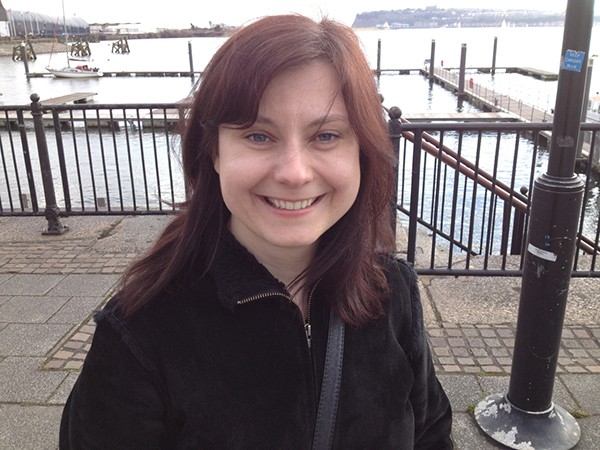
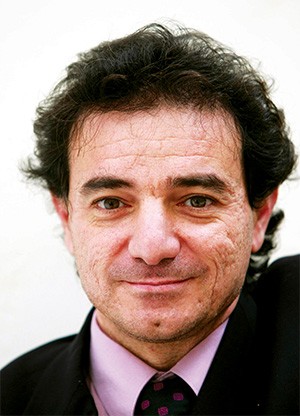
After the images have been labeled, the algorithm can draw the boundaries between digestive organs. With the boundaries in place, the specialist can focus on the small intestine. At the press of a button countless hours and cash are saved.
The software is still at the research stage. That research needs to eventually be turned into a software package for a hospital’s day-to-day examinations. In the future, the algorithm could possibly be inserted directly onto the capsule. An intelligent capsule would be born creating a recording process capable of adapting to the needs of the doctor. It would show them just what they want to see.
Ideally the doctor would have it even easier with the software highlighting diseased areas automatically. The researchers at the University of Malta want to start automatically detecting abnormal conditions and pathologies within the digestive tract. For the specialist, it cannot get better than this.
The result? A shorter and more efficient screening process that could turn capsule endoscopy into an easily accessible and routine examination. Shorter specialist screening times would bring down costs in the private sector and lessen the burden on public health systems. Michael would not need to worry any longer; he’d just pop a pill.
* Michael is a fictitious character
[ct_divider]
The author thanks Prof. Thomas Attard and Joe Garzia. The research work is funded by the Strategic Educational Pathways Scholarship (Malta). The scholarship is part-financed by the European Union — European Social Fund (ESF) under Operational Programme II — Cohesion Policy 2007–2013, ‘Empowering People for More Jobs and a Better Quality of Life’
Discovering Depression Treatments
Over 100 million people suffer from depression. Prof. Giuseppe Di Giovanni talks about his life’s work on the brain chemical serotonin to find a new treatment for this debilitating disease that touches so many of us
The winter rays of sunlight reflected off the snow upon Mount Maiella and the beautiful Adriatic Sea. They lit up the room where I was sitting with Dr Ennio Esposito (head of the Neurophysiology unit, Mario Negri Sud, Italy). On this cold day in February the light was blinding and it was difficult to make out my long time friend and colleague. Together we had studied the brain chemicals serotonin and dopamine vital for love, pleasure, addiction, and linked to depression — my research subject.
‘Ennio, I am tired and frustrated, I am increasingly convinced that our in vivo (whole organism) experimental approach is not the right one. There is too much variability in the results and if we really want to understand the cause of depression and find a new cure we need to get some reproducible data and change our tactic.’
“We still do not understand how many psychoactive drugs actually work, meaning that more research is needed”
At that time, I was using glass electrodes to study changes in the electrical activity of single neurons in brains. Additionally, I used a technique (microiontophoresis) that registers neuron electrical activity and also applies a very small amount of the drug. In this way, I could see which brain cell was active and how different chemicals might influence it. Surprisingly, though introduced in the 1950s, these techniques are still some of the best ways to study drug effects on a living brain.
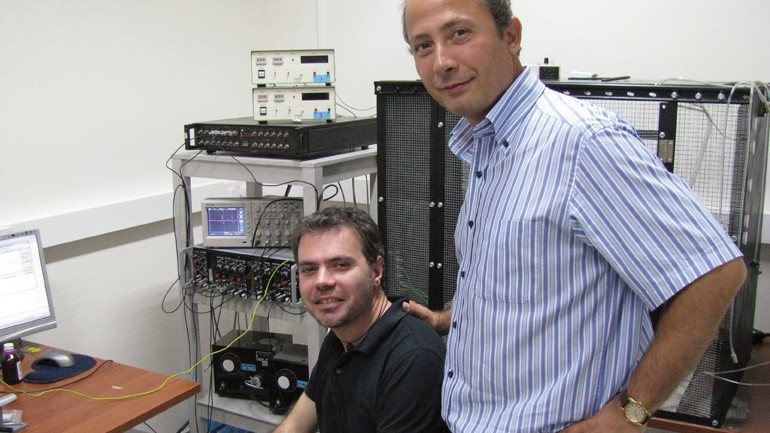
My research focuses on the role of two brain chemicals, dopamine and serotonin, in mental disorders. When stimulated neurons release chemicals (neurotransmitters). I am interested in dopaminergic neurons which release dopamine and serotonergic neurons that release serotonin. Once released, chemicals can pass through the spaces in between neurons and bind to another neuron stimulating or inhibiting it. They bind on proteins called receptors. When they do, they trigger the cell to fire or shut down. By triggering certain neurons in our brains, they reinforce or change our behaviour.
Dopamine is involved in the pleasure pathway. It switches on for behaviours like emotional responses, locomotion, and reinforcing good feelings. Changes in the level of dopamine effect a person’s reward and curiosity-seeking behaviour, like sex and addictive drugs. On the other hand, serotonin seems to have a more subtle role. One of serotonin’s major roles is to modulate or control the effects of other neurotransmitters, such as dopamine. In the words of Carew, a Yale researcher, ‘Serotonin is only one of the molecules in the orchestra. But rather than being the trumpet or the cello player, it’s the band leader who choreographs the output of the brain.’ The belief that serotonin is the brain’s ‘happy chemical’, that low serotonin levels cause depression and antidepressants work by boosting it is a very simplistic view. In truth, no one knows exactly how dopamine and serotonin levels induce depression.
“I have spent my life trying to figure out the role of dopamine and serotonin in the brain”
A lot of what we do know is because of animal research. The animals used to model this disease are given antidepressants to try and understand how effective they are and how they work. By studying their brains we can start to comprehend what causes depression. Right now we do not understand the whole picture behind the causes of depression and patients end up receiving inadequate treatment. We still do not understand how many psychoactive drugs actually work, meaning that more research is needed.
Most drugs were discovered by chance while being used to treat other disorders. For example, the antidepressant Iproniazid was originally developed to fight tuberculosis.
After the researchers saw less depression in patients suffering from tuberculosis they started prescribing it to depressed patients. In another example from the 1950s, clinicians discovered the first tricyclic antidepressant while searching for new drugs against other mental diseases.
Today, we fortunately have a battery of drugs that can treat depression. Unfortunately, the best drugs on the market only completely alleviate symptoms in 35 to 40 percent of patients compared to 15 to 20 percent taking a placebo (a sugar pill), a fact not publicised in pharmaceutical ads. Another problem is that when people begin taking antidepressants, mood changes can take four weeks or more to appear. This delay in action is one of the major limitations of these medications since it prolongs the impairments associated with depression, increases the risk of suicide, the probability that a patient stops treatment, and medical costs. To tackle these problems pharmaceutical companies and academic researchers want to find more effective and faster acting antidepressant drugs.
Ennio and I, together with Vincenzo Di Matteo and other researchers at the Mario Negri have tried to resolve the antidepressant lag time enigma by studying rats. We first inhibited the levels of serotonin for 3 weeks using the latest Selective Serotonin Reuptake Inhibitors (SSRIs) named fluoxetine, sertraline, and citalopram. Then we measured the electrical activity of dopamine and serotonin neurons in rat brains. We discovered that the therapeutic effect of antidepressants is not only due to their capacity to restore a normal level of serotonin activity. It also induces adaptive mechanisms in the dopaminergic system (that releases dopamine) because of repeated treatment.
How do SSRI’s treat depression? At first, these chemicals only slightly stimulate serotonin release. Long-term treatment kicks in an adaptive process. The receptor type located on serotonergic neurons which inhibit serotonin activity become insensitive. Repeated treatment frees serotonin neurons from this ‘brake’. By repeatedly using these drugs (with a lag time of 2–8 weeks), the levels of serotonin being transmitted increase and stay high for a longer time which is responsible for the SSRIs antidepressive effect.
The perfect antidepressant could lie in blocking the activity of these receptors since there would be no major delay in action. This hypothesis was confirmed by Francesc Artigas and his research group (University of Barcelona). They administered pindololo, a drug capable of blocking these serotonin receptors, and observed an increase of the antidepressive effect of the drugs paroxetine and fluvoxamine. They worked by reducing the latency period. Patients on pindololo did noticeably better and the clinical data matched that from laboratory animals. Blocking this type of serotonin receptors can be a promising therapy to reduce the latency period and possibly, increase antidepressant action.
My colleagues and I formed an alternative hypothesis as to why the clinical effects of drugs are delayed for so long focusing our attention on the dopaminergic system. We showed that acute administration of different SSRIs reduces the electrical activity of dopaminergic neurons, which release dopamine. These drugs increase the levels of serotonin, which decrease dopaminergic neuronal activity (which release dopamine) by over stimulating another inhibitory serotonin receptor this time located on dopaminergic cells. The result? The drugs taken to cure depression paradoxically initially induce a reduction of dopamine, which is meant to be the neurotransmitter of well-being and happiness! Indeed, SSRIs can worsen the depression of patients in the first few weeks of treatment.
When the drugs are used over a long period of time (3–4 weeks), the initial reduction of dopamine reverses. The change happens because the repeated treatment reduces the sensitivity of this type of serotonin receptor on dopaminergic cells freeing them from their serotonin ‘brake’.
“All of our work has made it possible to consider new treatments of depression”
We think we have found the reason why SSRI antidepressants take so long to work. Two different serotonin receptors need to become insensitive to the level of serotonin in the brain, one found on serotonergic cells, the other on dopaminergic cells. Their insensitivity allows the activity of dopaminergic neurons to return to normal even though the serotonin activity has been bumped up.
Other labs have confirmed our results, which is vital step for a theory to become fact. Cremer and his team (University of Groningen, Netherlands) have shown that blocking the same type of serotonin receptor on dopaminergic cells in rats can improve the effect of SSRIs antidepressants. Ultimately all of our work has made it possible to consider new treatments of depression, which I am very happy to see.
Many questions remain unanswered about depression. The most urgent task is to find a more effective way to treat it. This is my goal, I have spent my life trying to figure out the role of dopamine and serotonin in the brain — with some notable successes. I hope to see the next generation of antidepressants which would improve the life of 121 million depression sufferers.
Ennio listened to me as I expressed my frustration after once again obtaining conflicting results in the laboratory. ‘Giuseppe’ he said ‘You are right, billions of neurons in our brain behave differently, but as Douglas Adams said, ‘If you try and take a cat apart to see how it works, the first thing you have on your hands is a nonworking cat. Life is a level of complexity that almost lies outside our vision’ (Hitchhikers Guide to the Galaxy). If we want to break the code of the brain and hope to treat its diseases we need to take a holistic approach that takes the whole brain into account.
[ct_divider]
Article dedicated to the prominent researcher Dr Ennio Esposito, Prof. Di Giovanni’s (Department of Physiology and Biochemistry, UoM) colleague and friend. In 2011, he died of a heart attack. During his last years, he suffered from a severe refractory bipolar depression. If interested in an M.Sc. or Ph.D. in biological psychiatry please contact Prof. Giuseppe Di Giovanni
Further Reading
Bortolato M., Pivac N., Muck Seler D., Nikolac Perkovic M., Pessia M., Di Giovanni G., (2013) The role of the serotonergic system at the interface of aggression and suicide. Neuroscience, 236:160-185.
Di Giovanni G., Esposito E. Di Matteo V., (2011). 5-HT2C Receptors in the Pathophysiology of CNS Disease. Springer, New York.
Di Giovanni G., Di Matteo V., Esposito E. (2008) Serotonin-Dopamine Interaction: Experimental and Therapeutic Evidence, Progress in Brain Research, 172. Elsevier, Netherlands.
Depression — The Dana Guide
Depression — National Institute of Mental Health, USA
TED talk about targeted psychiatric medications, similarly to Prof. Digiovanni borne on the realisation that current treatments are not good enough for everyone