What is the universe made of? How was it formed? How old is it? Will things stay the same forever?
The night sky is shedding its secrets as Ian Fenech Conti (Institute of Space Science and Astronomy) talks about his work measuring the most elusive matter in the universe.
Till recently, our curiosity about the cosmos could only be mitigated by our imagination. Everything changed in the past century with the development of powerful telescopes that can peer into the early beginning of the Universe. Today’s telescopes are starting to uncover its deepest and darkest corners.
Astrophysicists are faced with a major problem. Most of the known Universe cannot be seen directly. If we had to categorise all things that have mass and energy in the entire Universe—normal matter, like planets, galaxies, stars, dust, rocks, and gas—they only make up about 4% of the entire Universe. So what is the other 96% of the Universe made up of? There is no simple answer, however our current understanding has unravelled another two ‘hidden’ parts of the puzzle. These are called dark matter and dark energy, and are so named because we are not exactly sure what they are. To make matters worse we cannot see them directly and can only tell that they exist through their effects and interactions with normal matter.
One of the interplays we study between the invisible dark matter and normal matter is called Gravitational Lensing. The principle is simple and we see the lensing effect all around us. People wearing prescription glasses use it all the time. Light bends as it passes through glass; large celestial bodies with a huge gravity can also bend light.
A Lens on the Universe
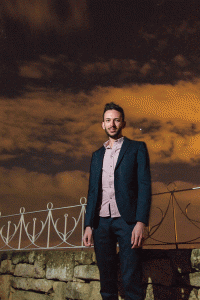
Gravitational lensing works similarly to prescription glasses but is an effect of Einstein’s theory of general relativity. All it means is that all matter, such as the Earth, stars, and galaxies, have mass and a gravitational field. The gravitational field of stars and galaxies causes light to bend as it passes close by. Naturally, the greater the mass of the object, the stronger the gravitational field and the more it causes the light to bend. Groups of galaxies clustered together end up acting like large lenses floating around in space, bending light as it passes by them. The lensing effect with stars and planets occurs also on smaller scales, for example around humans, but the bending effect is too small to measure.
In order to understand what dark matter is we need to look at the gravitational lensing effect on the largest of scales: galaxies and clusters of galaxies. Using modern telescopes, we take incredible images of the night sky that reveal thousands of galaxies. Every light ray that each one of those galaxies emitted had to pass through the Universe, sometimes for millions of light years, ending up all the way on Earth, inside our telescopes to form an image. On its way to us, the light ray had to pass by other galaxies and clusters which cause it to bend. However most of the bending or lensing effect is not an effect of normal matter but dark matter, that invisible stuff that contributes a huge 85% of the Universe’s mass.
“Groups of galaxies clustered together end up acting like large lenses floating around in space, bending light as it passes by them”
Dark matter is located wherever normal matter is, this means that it lies » around and between galaxies and when we have a large collection of galaxies next to each other—a galaxy cluster, most of the mass comes from dark matter. The light passing by or through that cluster is lensed, predominantly due to the quantity of dark matter present. The amount of dark matter is not uniform in any given area, this means the lensing effect can be very strong in some places and very weak in others. So much so that when we look at the images we take of galaxies with our telescopes, some of them appear to have arced, stretched and disfigured shapes. This does not mean that the galaxy actually looks like that, but that the light ray was heavily lensed on its path to us, giving us a warped representation of what is really out there.
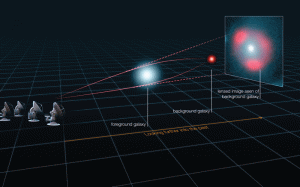
The shape distortion of galaxies happens because a galaxy is a very large object. Light rays that are emitted from one side of the galaxy pass through different regions of space than light that is emitted from the other side and it is the culmination of all this light bending that produces the final distorted shape we see. The gravitational lensing effect can sometimes be so strong that it produces multiple images of the same galaxy. Another interesting by-product of gravitational lensing is that it can act as a magnifying glass, increasing the visibility of distant galaxies that we would not have been able to see otherwise.
In these images, when we clearly see the galaxy shape distortions with just our eyes, the lensing strength is considered very strong. This means objects with a very large mass, such as a supercluster of galaxies, fell between the galaxy and us, causing the image we see of the galaxy to be very distorted. Here it is relatively easy to measure the lensing effect. The reality is that there aren’t that many superclusters capable of producing strong lensing effects. Strong lensing is a very rare occurrence.
In order to realistically measure the dark matter distribution we need to be able to measure the weaker variation of gravitational lensing, which is significantly more common. Since normal matter and dark matter almost always coexist, it implies that to some degree a galaxy image we see from our telescopes is always partly lensed by dark matter. This mild variant of gravitational lensing is called weak lensing and only causes slight shape distortions of about 1%.
Measuring these minor shape distortions would allow us to map and understand the way dark matter is distributed throughout the Universe. The major problem here is that these variations are so weak that it is impossible to see and measure them with the naked eye. The key to all this is the ability to develop an accurate technique capable of measuring these slight variations.
In practice, we do not need to know how much every single galaxy is lensed. The lensing effect can be averaged across a set of galaxies within the same neighbourhood. Of course, doing so means making a number of assumptions. The first assumption is that all galaxies tend be elliptical in their overall shape and the second one is that they all have random orientations in the sky. Once we take these assumptions into consideration, we can start to apply statistical techniques that give us a good indication of the dark matter distribution for any given patch of sky.
Since we are dealing with such high levels of sensitivity it is crucial that the techniques we develop are robust and accurate. When we take an image of the sky there are a lot of external factors that make the processes of measuring the galaxy shapes much harder. Apart from gravitational lensing, a ray of light is also ‘offset’ by a number of secondary effects as it enters Earth and hits our telescopes. There are three main secondary effects, the first occurs when the light enters our atmosphere, here the light ray is refracted and bounces all over the place; the second is the effect of the telescope mirror that smoothens the image causing a slight loss in detail and information; the third is the effect of camera-shake, since a typical astronomical image is taken with a long 2-minute exposure, any camera movement causes blurring. These result in a ‘dirty’ image that needs refining.
Malta: cleaning up space images
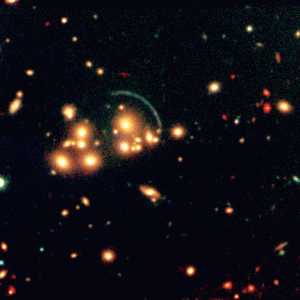
Dark matter usually only contributes to a minute 1% shape change so images need delicate treatment to clean up accurately since a dirty image is useless. For an image to be useful it must only be distorted due to the gravitational lens and nothing else. Thus various techniques are needed to remove these secondary effects. This is one of the problems that we try to tackle at the Institute of Space Sciences and Astronomy (University of Malta). How do we go about removing these effects? First, we start off with an image that contains a large number of galaxies and stars taken by a telescope pointing somewhere at the night sky. We know that all the galaxies we see in the image are distant objects, meaning they have been subject to some amount of gravitational lensing. However the lensing signal is hidden behind the secondary effects, so the next challenge involves finding a way to separate the two.
While galaxies suffer both lensing and secondary effects, stars in the same image do not. Stars are much smaller than galaxies, being smaller means they emit less light and so they must be much closer to us than the galaxies. Visible stars come from our own galaxy: The Milky Way. These stars are too small and close to us to be affected by the gravitational lensing, yet are still affected by secondary effects. The stars in an image can be studied to determine which distortions are due to secondary effects and which due to gravitational lensing.
To speed things up, computer algorithms are used to automatically select stars. The program relies on the following assumptions. Firstly, that stars tend to be relatively bright and secondly, that light from stars is concentrated at a central peak. Stars are bright at the centre with very narrow edges, while a galaxy is less bright at the core and has broad edges of light. This classification technique separates the image into stars or galaxies.
Once we have extracted a catalogue of stars in our image, what’s next? Stars are usually circular or elliptical in shape, so if we look at the stars and see that they are a different shape we know that in that area of the image there are certain secondary effects and distortions. The amount of secondary effects is not uniform and an image is degraded by different amounts and directions across it—called spatial variation. A good image needs a large number of spread out stars to model the degradation properly.
Using the stars as sampling points of the secondary effects, our algorithm then begins to look at each star’s degraded shape and model it. The degraded stars we see in the images have complex shapes and it turns out that selection of the correct model is a very important choice. The approach we use is not constrained meaning that a degraded star shape can be virtually anything, from a simple elliptical shape to very complex geometric ones. This should allow the algorithm to make an accurate model of the way the secondary effects are distorting light in the image.
We now have a model of what the secondary effects look like at each star position. To remove these secondary effects from the galaxies in the image we have developed an algorithm that takes what we learnt above about how the stars are being distorted and applies them to the galaxies. Another triangulation algorithm figures out which of the nearby stars are best to use to understand the correct shape of the galaxy.
The algorithm then makes use of principal component analysis, artificial neural networks, and genetic algorithms to give us a best guess result on what we think the secondary effects are throughout the image. At this point we are able to remove all the secondary effects on any galaxy anywhere in the image. This process is repeated for each of the galaxies in the image, finally leaving us with an image containing clean galaxies, affected only by the gravitational lensing effect.
We only contribute to a small part of a long and complex process that ultimately ends up with… what? The truth is that we are not quite sure yet. We are trying to quantify something we do not quite understand. When we look at the way the Universe works when applied to the current models, things do not add up. It forces us to ask questions like “why do we get these weird galaxy shapes in our images?” and “why is the Universe expanding as it is?” By better understanding dark matter, we can answer these questions about the Universe that ultimately shed light on where we all come from.•
Comments are closed for this article!