Under Europe lies a 27 km tunnel that is both the coldest and hottest place on Earth. The Large Hadron Collider (LHC) has already found out what gives mass to all the matter in the Universe. It is now trying to go even deeper into what makes up everything we see around us. Dr Marija Cauchi writes about her research that helped protect this atom smasher from itself. Photography by Jean Claude Vancell.
It was 5 a.m. on the 4th of July 2012. I was in the middle of a long queue of scientists and engineers, all eagerly waiting to get into the auditorium at The European Organisation for Nuclear Research (CERN), near Geneva, Switzerland. Scientists worldwide had been waiting for this day for decades. The vibe outside the auditorium was incredible; we were bustling with excitement. A flurry of rumours, leaks, and hype otver the past days nodded towards humanity having found the Higgs Boson—the world’s most-wanted and long-awaited particle.
Being at CERN for my Ph.D. studies (as part of an ongoing collaboration between CERN and the University of Malta), I certainly could not miss this once-in-a-lifetime event. The queue of people built up quickly, and after 7 a.m. people were being turned away due to limited space in the auditorium. Time seemed to crawl by, but with the first rays of sunlight we knew the doors would open soon. We were finally seated by 8.30 a.m.
Everything around us is made up of a few basic building blocks called fundamental particles. How these particles work and stick together is through so-called fundamental forces. The Standard Model describes how these particles and forces behave together, which basically defines how the world around us works. This model is currently the best description of the subatomic world, but it is still like an incomplete jigsaw puzzle.
The Large Hadron Collider (LHC) at CERN is running experiments to help find the missing pieces. One of the missing pieces was identifying which particle is responsible for mass in all matter. Think about what determines a person’s weight. That is a combination of the mass in a person together with the force of gravity. The next question is what determines a person’s mass. And that was the answer given by the Higgs Boson particle, hence the huge fuss upon its discovery.
The famous Higgs Boson
The Higgs Boson is an elementary particle. This particle works with what is called the Higgs field. Imagine a political mass meeting taking place at the Granaries in Floriana. A common person would wander through the crowd with ease. This situation would definitely change on the arrival of the party leader. Fans would start clustering around, trying to shake hands and in the process making his/her passage more difficult as s/he tries to make it to the podium. These people would represent the Higgs field. They would slow down the party leader. This impediment is like a particle having a large mass which has to work harder to get through the Higgs field.
Coming back to the political meeting, imagine a situation in which a rumour spreads that the party leader is going to announce an update on a hot political topic. This rumour would move from one end of the political meeting to the other. The rumour works in a similar way to how the Higgs Boson excites the Higgs field and moves through it.
The matter-microscope
The LHC is an underground 27 km ring located under the Franco-Swiss border near Geneva. It was finished in 2008, cost around €5.8 billion and operates by sending two beams of subatomic particles spinning in opposite directions around the ring. Once these beams come close to the speed of light, the machine smashes them together. At the designed full energy of 7 TeV per beam, each of the two beams has a total energy equivalent of a French TGV train travelling at 150 km/h, enough energy to melt 500 kg of copper.
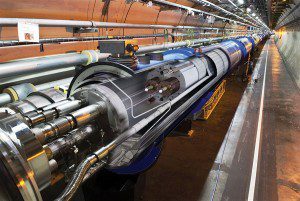
The beams are collided at four different points. These represent the main physics experiments or detectors. For just a moment, the collisions generate temperatures around 100,000 times hotter than the Sun, replicating the conditions that prevailed just after the Big Bang that created the Universe 13.82 billion years ago. The detectors give us a 3D image of the subatomic particles hurled out from the particles’ destruction. These traces are then closely analysed for movements, properties, or novel particles that could advance our understanding of matter. The LHC has multiple detectors to observe the same phenomena multiple times—an essential part of scientific rigour. ATLAS is one of the main LHC experiments and it generates data at the same rate as if everyone on Earth were to simultaneously make ten phone calls each.
A Proper Job
The LHC has an essential collimation system. A collimator is meant to trim beams to remove particles that have gone slightly off-track. The collimator plays an important role in preventing these particles from getting lost. If they do get lost, they can cause damage to the beam pipe and the superconducting magnets that need to be kept at temperatures colder than space itself. There are over 100 collimators installed around the ring. Each collimator consists of two parallel jaws, with the beam passing through the centre of the jaw gap. Being so close to the beam makes the collimators rather radioactive.
When I was at CERN, I used to help set up the collimation system. This took place at the CERN Control Centre—the heart of the experiment. My responsibility was to support the team in the collimator alignment before the beams could circulate in the LHC. I spent many nights at the CERN Control Centre but the friendly and exciting atmosphere always made it more than worthwhile. The only thing I dreaded was hearing ‘Dump Beam 1, Dump Beam 2’. Nobody liked hearing this command as it meant something had gone wrong and other magnets would have extracted the beams out of the LHC to prevent damage. This also meant that we would have to wait for a couple of hours until the LHC operators and engineers would re-inject the beams and need us to realign the collimators.
The collimators are a key example of vital equipment that needs precision engineering. They need to be resistant enough to protect the LHC from the destructive beam in case of an accident. Dedicated mechanical engineers at CERN need to ensure that the collimators’ design is robust enough to withstand beam accidents without compromising what they do to keep the beams circling smoothly. My Ph.D. focused on the performance of collimators in such situations.
Surely Nothing can go wrong?
If the beam alignment is inaccurate, the beam can smack on the face of a collimator jaw. Known as a beam accident scenario, it can cause the same damage as 80 kg of TNT explosive. Such an impact can quickly raise the jaw’s temperature by thousands of degrees and melt the jaw, leaving it permanently damaged.
“Collimators are expensive. Each one costs around €127,000. Experiments cannot test every scenario where things can go wrong in the real world. Computer models are needed to crash test the collimators.”
Collimators are expensive. Each one costs around €127,000. Experiments cannot test every scenario where things can go wrong in the real world. Computer models are needed to crash test the collimators. Advanced simulations are part and parcel of state-of-the-art engineering work, enabling highly specialised technical people to design systems at a level that was unimaginable some decades ago.
A model collimator
After familiarising myself with the collimation system, I needed to come up with a working model of the tertiary collimator—one of the most important. The complexity of the collimator jaw made this a very challenging task. I managed to develop the model over several weeks involving numerous iterations and lots of parameter tweaking to get it right. Finally, it worked. The next step was to identify new ways the beam could fail. These scenarios were based on accelerator physics studies by the collimation team at CERN.
Crash Test Buggy
The collimator jaws are usually set up to be in perfect alignment with the beam. So what happens if the jaws are not perfectly aligned and beam impact occurs? The collimator jaws’ inclination can be manipulated, and I started varying their tilt to try and find an answer.
The jaw angle did affect the heat distribution. At an angle of 0.06o, I saw a remarkable change in temperature distribution along the collimator’s length. Such a jaw inclination lowered the overall peak temperature caused by the beam impact, hence mitigating the induced damage.
“I vividly remember taking a look inside the collimator tank. I was stunned when I saw the destructive potential of the LHC beams.”
The LHC’s beam is made up of either protons or heavy lead ions. These behave differently upon interaction with the collimator jaws but they had never been compared upon impact on the collimator. While investigating this problem, I kept all simulation parameters constant and only changed the particle type (proton or lead) to maintain a good comparison. Although the particles behave differently, no major discrepancies were observed and the collimator responded similarly to both beam types.
Going live
Any computer model needs to be tested in the real world to make sure it actually works. The collimator model was validated through a first-of-its-kind experiment at CERN. An extensive campaign that needed months of preparation to extract the most complete information from the test was set up. It was the only test I would perform during my stay at CERN to reproduce LHC accident scenarios by purposely shooting the beam on a real collimator. Sensors for jaw temperature, water temperature, water pressure, and vacuum pressure, as well as microphones to “listen” to the beam, were installed to get the most from this almost €200,000 experiment.
The tests were performed over a number of days because of beam preparation time. We checked everything over and over again to avoid any mistakes. The data obtained was priceless.
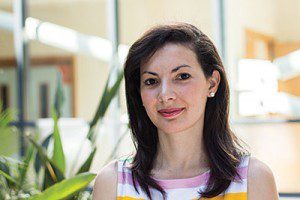
The model did not always match reality. When I plotted the jaw temperature, I saw an increase of only a few degrees upon beam impact. I was bitterly disappointed. I knew that a much higher temperature increase was expected. What could have happened? While troubleshooting, I realised that the contact pressure between the sensor and the back of the jaw might have been weak. The computer simulations confirmed this. Everyone was surprised. This meant that the jaw temperature sensors on the LHC collimators were fitted improperly, leading to unreliable temperature recordings. Because of this observation, new temperature sensor fixation methods needed to be designed and were implemented on the new collimators installed earlier this year.
Another highlight was opening the tested collimator tank. This needed four months to cool off to safe radiation levels. I vividly remember taking a look inside the collimator tank. I was stunned when I saw the destructive potential of the LHC beams. The beam had cut deep grooves on the jaw surfaces and blew material all around the tank. Happily, these observations matched my simulations and proved that the computer model worked correctly.
The announcement
‘I think we have it,’ said Rolf Heuer, CERN director general at the time. We had found the Higgs Boson. Applause, whistles, cheers, and camera flashes filled the auditorium, as this announcement circled the globe. Many years of work and volumes of collected data made us very confident of the discovery. This announcement led to a Nobel Prize in Physics in 2013.
Peter W. Higgs, who had predicted the particle, shed tears of joy inside the CERN auditorium. I had goose bumps all over me. Despite the exhaustion and lack of sleep, I headed towards my office, more determined than ever to make a difference with my discoveries.
This Ph.D. research was supervised by Dr Ralph W. Assmann (CERN, DESY), Dr Ing. Pierluigi Mollicone (University of Malta), and Dr Ing. Nicholas Sammut (University of Malta). It was carried out within the Department of Mechanical Engineering (University of Malta) and the Beams Department (CERN). It was supported by CERN, the University of Malta, and the EC FP7 Research Infrastructures projects EuCARD and EuCARD-2.
Further reading
- M. Cauchi et al., High energy beam impact tests on a LHC tertiary collimator at the CERN high-radiation to materials facility, Phys. Rev. ST Accel. Beams, 17:021004, February 2014.
- M. Cauchi et al., Thermomechanical assessment of the effects of a jaw-beam angle during beam impact on Large Hadron Collider collimators, Phys. Rev. ST Accel. Beams, 18:021001, February 2015.
- M. Cauchi et al., Thermomechanical response of Large Hadron Collider collimators to proton and ion beam impacts, Phys. Rev. ST Accel. Beams, 18:041002, April 2015.
Comments are closed for this article!