Better recovery for patients, reduced need for revision surgeries, and many hundreds of thousands of euros saved for public health and industry. That could be the outcome of four years’ intense work by engineers and medical professionals at the University of Malta and Mater Dei Hospital in developing biodegradable metal-based tailor-made bone scaffolds. Cassi Camilleri writes.
Healthy bones matter. They carry us through life, literally.
But we all know that journey can be fraught with difficulties. Cancer, a bad fall, even the simple passage of time, all of it impacts our bones. And not in a good way.
As a result, more and more people are visiting their orthopedic department at hospital, and more and more people are needing corrective procedures that involve bone implants or grafts. According to research published in 2017 by Wang and Yeung , that number lies at around 2.2 million procedures worldwide.
The status quo, its flaws, and the need it harbours
‘If we need to repair bone, the first option is an autologous bone graft,’ explains orthopedic surgeon Ray Gatt. With these autografts, surgeons fix the damaged area by harvesting bone from another part of the patient’s own body. While this method has obvious pros in biocompatibility, it also has cons.
‘For the patient, it means you’re giving them one incision where the primary operation is and then another incision where you’re taking bone from. So they’re effectively having two surgeries. Occasionally, that second incision is much more painful than the first.’
The extent of the damage in question is another limitation to the autograft. ‘You can only harvest so much bone from a patient’s own body. It’s a finite resource,’ explains Gatt. If a bone has multiple fractures from a car accident, for example, surgeons would then turn to a combination of bone graft substitutes and permanent implants.
These require weeks of downtime from the patient until the grafts consolidate and can bear weight on them. However, there are other issues that Gatt’s colleague Ryan Giordmaina highlights: ‘In big defects, you want to give the bone strength and support; however, when you’re doing this, you’re also taking away the bone’s ability to regrow and reform.’
Bone has a funny way of regenerating. Researcher Prof. Pierre Schembri-Wismayer explains it this way, ‘When you break a leg, surgeons put a cast on and ask you to rest for a while, then when the bone has formed a bit, you progress to weight bearing, and it’s time to start walking. The reason is that bones can feel stress passing through them and rebuild themselves according to that stress. It’s an amazing capability.’
Plates and other hardware that are implanted pose the same issue. ‘Plates carry a lot of their weight themselves,’ continues Giordmaina. ‘So the force isn’t passing through the bone, and to some extent, there can be some loss of function. This is why the current drive in revision surgery is to have more bone biology. You want the bone to grow back as much as possible.’
To make this happen, the medical world needs an implant that ticks a number of boxes. It has to be strong and mechanically sound. But it also needs to be porous to allow for vascularisation and nutrients to pass through. It needs to be biodegradable so the patient’s own bone could grow back, but the implant and the degradation material both need to be biocompatible and non-toxic to the patient.
It’s a tall order, with a lot of conflicting needs at the outset. But that was the brief. And that was what the engineers needed.
With that, and continued support from Schembri-Wismayer, Gatt, Giordmaina, and many others at the University of Malta, Knowledge Transfer Office, Project Support Office, and Mater Dei Hospital, as well as the Malta Council for Science and Technology, Prof. Joseph Buhagiar kicked off the BioSA project.
Early days and leaving the known behind
This particularly complicated problem-solving process started with Buhagiar and his then-student, now collaborator Christabelle Tonna experimenting with powdered metals. They wanted to replicate the net-like structure of spongy bone, and this is why they use actual, off-the-shelf washing up sponges as a way to create metal templates.
Using different powdered metals and a slurry liquid to bind it together, they covered the sponges and then applied heat. This fuses the powdered metals together in a process called sintering, while burning the sponge off at the same time. This is what’s called the replication method, and it worked. ‘In the end, we were left with a scaffold of sorts,’ Buhagiar confirms.
As expected from first drafts, it wasn’t perfect. ‘Among other things, the scaffold wasn’t reticulated like real bone is,’ Buhagiar explains. ‘There was a network, sure, but there were too many dead ends. Real bone isn’t like that, so we needed to find another way. But it was a start. A proof of concept we would continue to build upon.’
And build they did.
In fact, the first few months of the project were dedicated to making leaps. The team wanted the scaffolds to be tailor-made to the patient, so the ready-made sponges had to be left behind. With Dr Arif Rochman’s help, the team looked into 3D printing solutions.
Together, they decided to use a stereolithography 3D printer. Unlike standard filament printers, its advanced resolution would allow them to print a plastic scaffold template with small enough gaps to allow the patient’s blood supply to flow through the implant. It would even allow them to create a gyroid structure for the template instead of the straight lines we usually see in scaffolds.
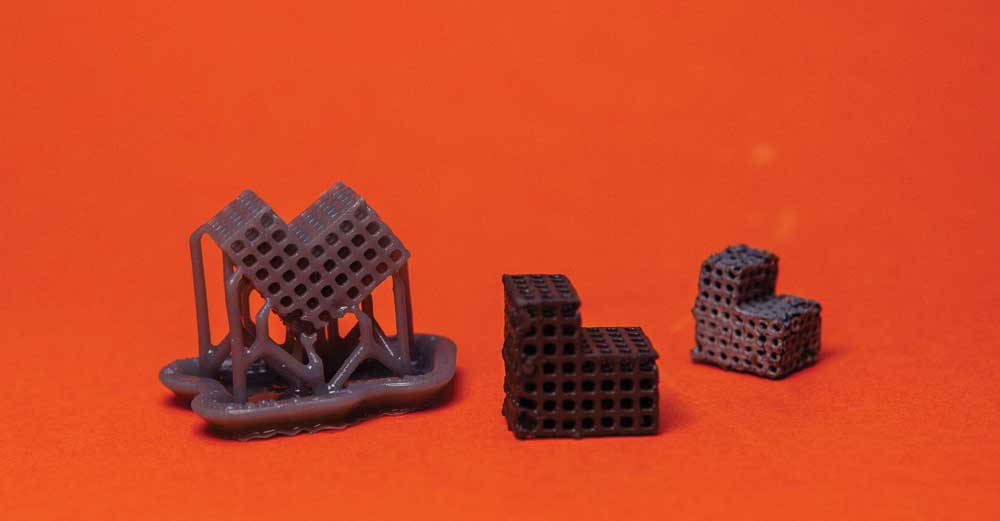
The stage of scaffold preparation-3D printed polymer template, metal coating the template and sintered scaffold (from left to right)
Photos by Elisa von Brockdorff
In another part of the engineering lab, collaborator Prof. Ing. Maurice Grech was helping Buhagiar run a project in parallel to find the right powdered metal recipe for the scaffolds. Yes, metals are the best for giving the scaffold the strength it needs to bear the body’s weight, but iron can also be toxic.
‘Iron is present in the body, but iron isn’t easily got rid of by the body,’ explains Schembri-Wismayer. ‘It stays stored, but the body can get overwhelmed. And if it does, iron damages the kidneys, heart, and liver and causes them to stop functioning. So we had to find a balance.’
This balance is the key to the whole project.
‘In engineering, we’re usually working on corrosion resistance, coatings for resistance. In real life, you want to protect the item from corrosion. All our knowledge is built around that. But with BioSA, it was about increasing the rate of corrosion. The work needed us to flip all our knowledge as engineers on its head,’ Tonna explains. ‘It was a massive paradigm shift,’ nods Buhagiar.
This mindset saw the team making more jumps as they went. Though the initial process included a binder to glue the powdered metal together, experimenting further with the polymer they were using for 3D printing brought about another option.
‘We noted a tackiness developing in the material depending on the curing we did,’ Buhagiar says, ‘So we used this tackiness on the printed template surface to adhere the metal powder directly.’
From there, they went on to develop a two-step heat treatment that would later be streamlined to one. It involves placing the plastic template covered in metal powder into a furnace. At 450°C, the polymer template burns away, and at 1120°C, sintering happens, and the powders join to form a solid implant.
Trials, tests, and some road ahead
The team is now in the process of running various tests on their new implants. Their first ‘patients’ were various pork bones bought from a butcher. The orthopaedic surgery trainees conducting the procedure, Luke Saliba and Keith Sammut, report that ‘the scaffolds were easy to handle and adjust as needed.’
Saliba is also working on cytotoxicity testing, which tells the team how the corroding metal in the implant is affecting live cells grown in a petri dish. The results of this work continue to help the team tweak and perfect the implant’s powder recipe.
Meanwhile, Sammut is conducting bacterial testing. ‘Whenever you’re implanting metal work in the body, there is a risk of infection,’ Sammut explains. ‘The problem is whenever you have an indwelling device like a metal rod or plate, bacteria tend to form a biofilm. Biofilm acts like a shield for bacteria.’ Worryingly, it makes bacteria resistant to antibiotics.
‘Antibiotics alone are not enough to tackle this problem when and if it happens, and it often necessitates the removal of the implant. An infection from a prosthetic device is devastating’.
To solve this problem, the team has added silver to their alloy. With it, ‘there was a reduction of 92 to 98% in the biofilms when we added silver,’ Sammut reports.
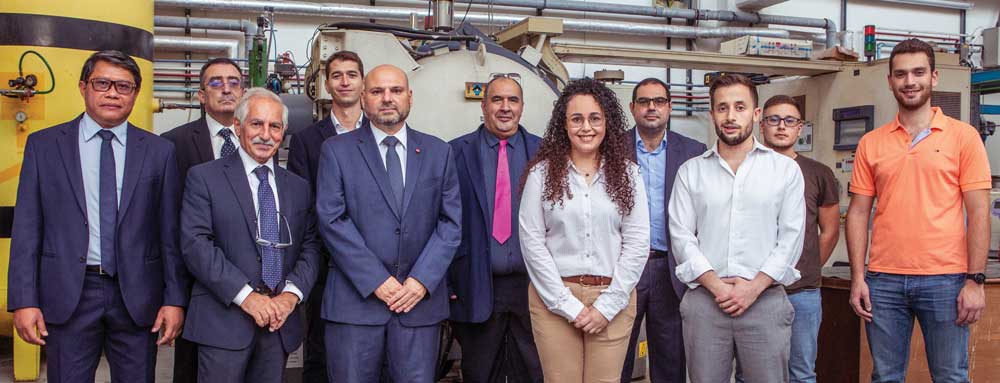
So what are the next steps for this project, you ask?
At this point, more and more energy is shifting into solidifying the business plan and giving the team the information they need to make informed decisions on the way forward. Crucial to this is the Knowledge Transfer Office (KTO) and Nicola Camilleri.
‘Our role as KTO is to complement the scientific team by bringing in the business mind to the development at a relatively early stage. Just like BioSA, every new invention has the potential of having a great business opportunity ingrained. However, this promising business case has to be validated and a business plan formulated, essentially alongside the technology development. So we have been working hand in hand. While the research team has been advancing the technology’s readiness, we have been advancing the market readiness of the future product. In this project, though we are still at the beginning, we just completed the filing of a patent application, which is one of the earliest possible steps on the business side.’
While this might seem like an anti-climax to some, this is the reality of product development, especially in the medical field.
‘The way our national health service operates is that it looks at what has been tried and tested all over the world,’ explains Giordmaina, ‘so a product like this will have to go through a period in the market throughout the world and be taken on by surgeons worldwide. Only then will it be considered to be incorporated in our practice. That’s how most implants nowadays are brought into our national health service.’
But this is no reason to despair, as Gatt explains. ‘It’s true, I probably won’t use the BioSA scaffolds in my surgical lifetime, but what Ryan described is a necessary process that should fall into place. The reality is that the idea is the most difficult part. Getting the conception of the technology. And I think, with BioSA, that has been done. The hard part has been done. Now, we wait for all of it to pay off.’
The ideal bone scaffold
Bone scaffolds are 3D structures, usually made of ceramic materials, used for bone reconstruction. The ideal bone scaffold should be:
- Porous to allow for vascularisation and transference of nutrients and waste
- Strong and mechanically sound
- Biodegradable
- Biocompatible and non-toxic
Contributing to Malta’s research future
The funds granted by the Malta Council for Science and Technology (MCST), a total of approximately €240,000, have been essential for the development of the BioSA scaffold as a product. They have also gone towards securing its business potential through market research, various drafts of business plans, and the patenting process.
Somewhat less obviously, MCST’s funding has also contributed to the development of Malta’s future researchers. ‘Christabelle Tonna’s PhD, as well as Luke Saliba and Keith Sammut’s MSc by Research were made possible through this fund,’ explains Buhagiar.
Gatt elaborates further: ‘With the research experience they have gained through the BioSA project, [Christabelle, Luke and Keith] have the tools they need to go forward, plan their own projects, and innovate themselves, creating solutions for more of the challenges we are facing in our fields.’
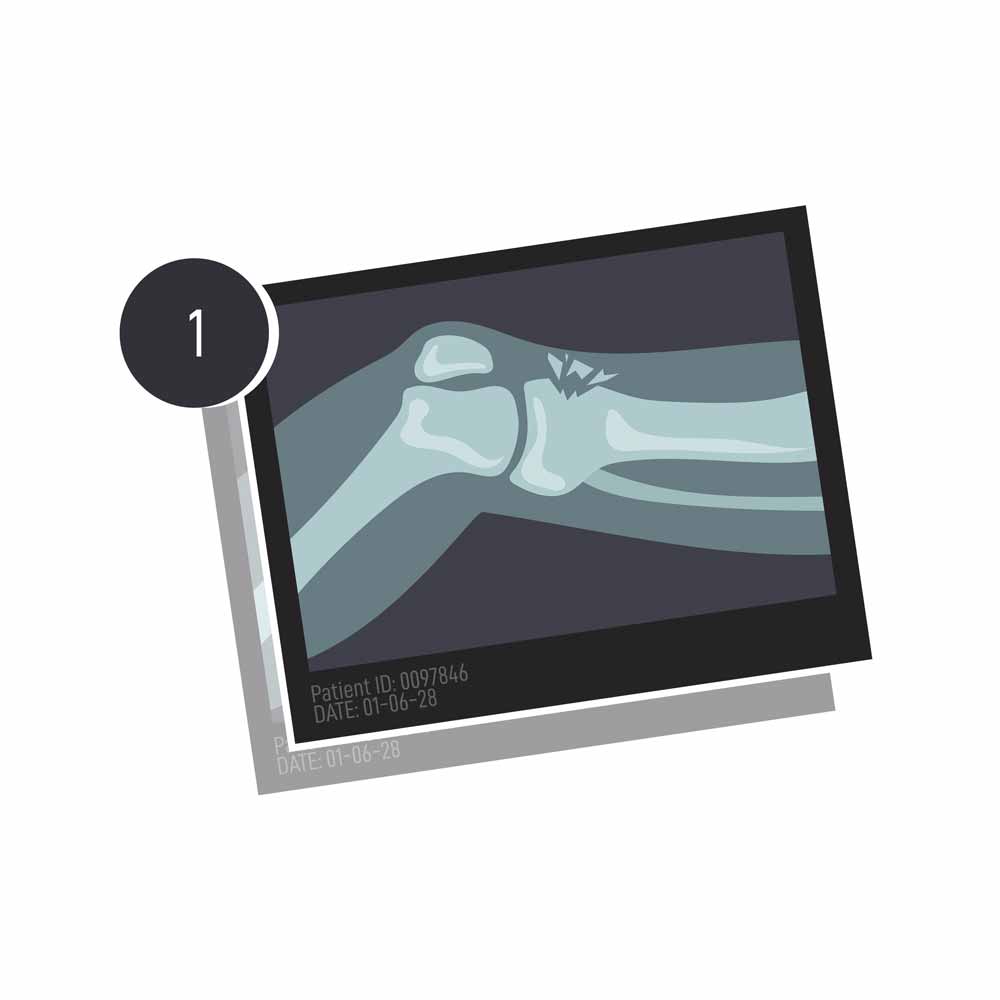
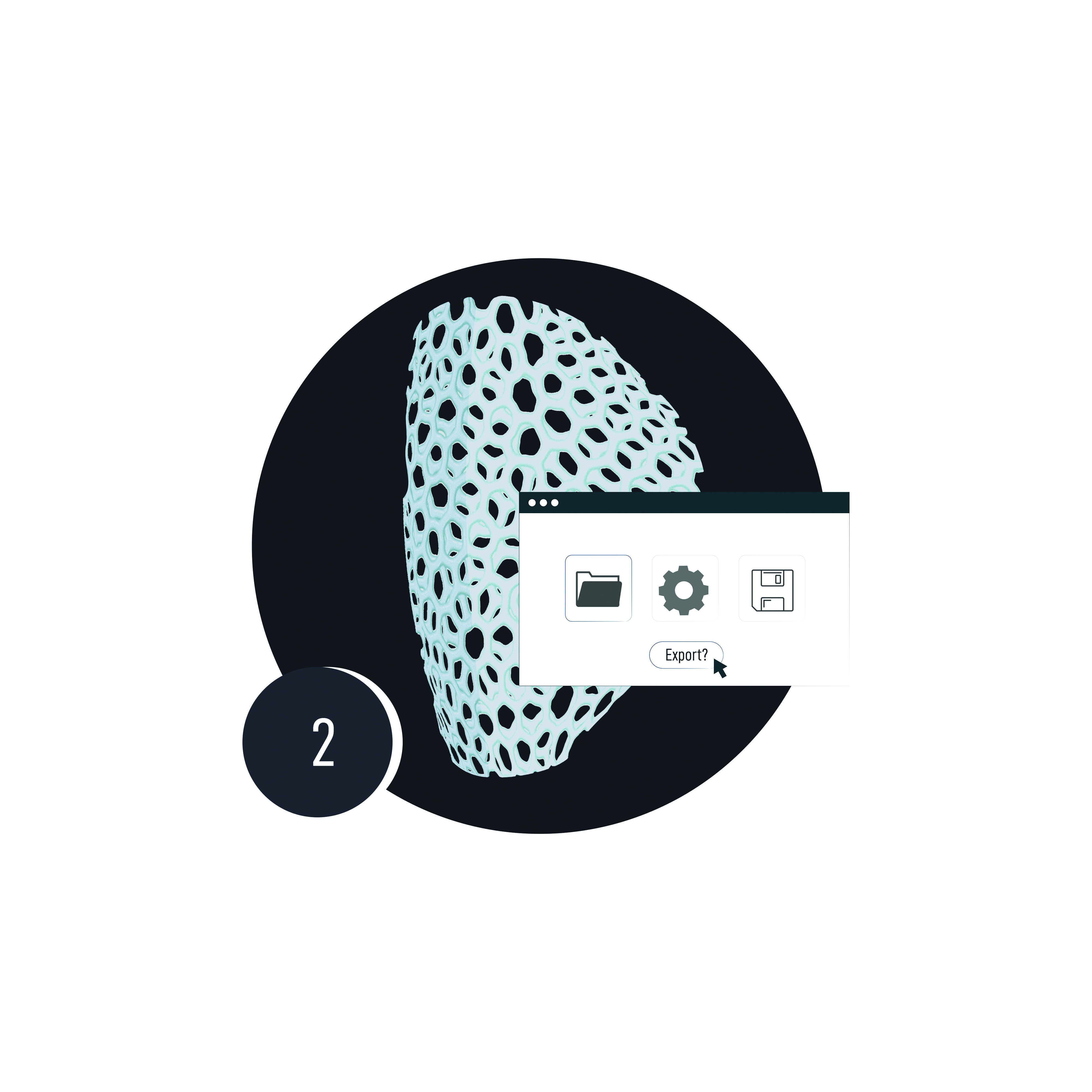
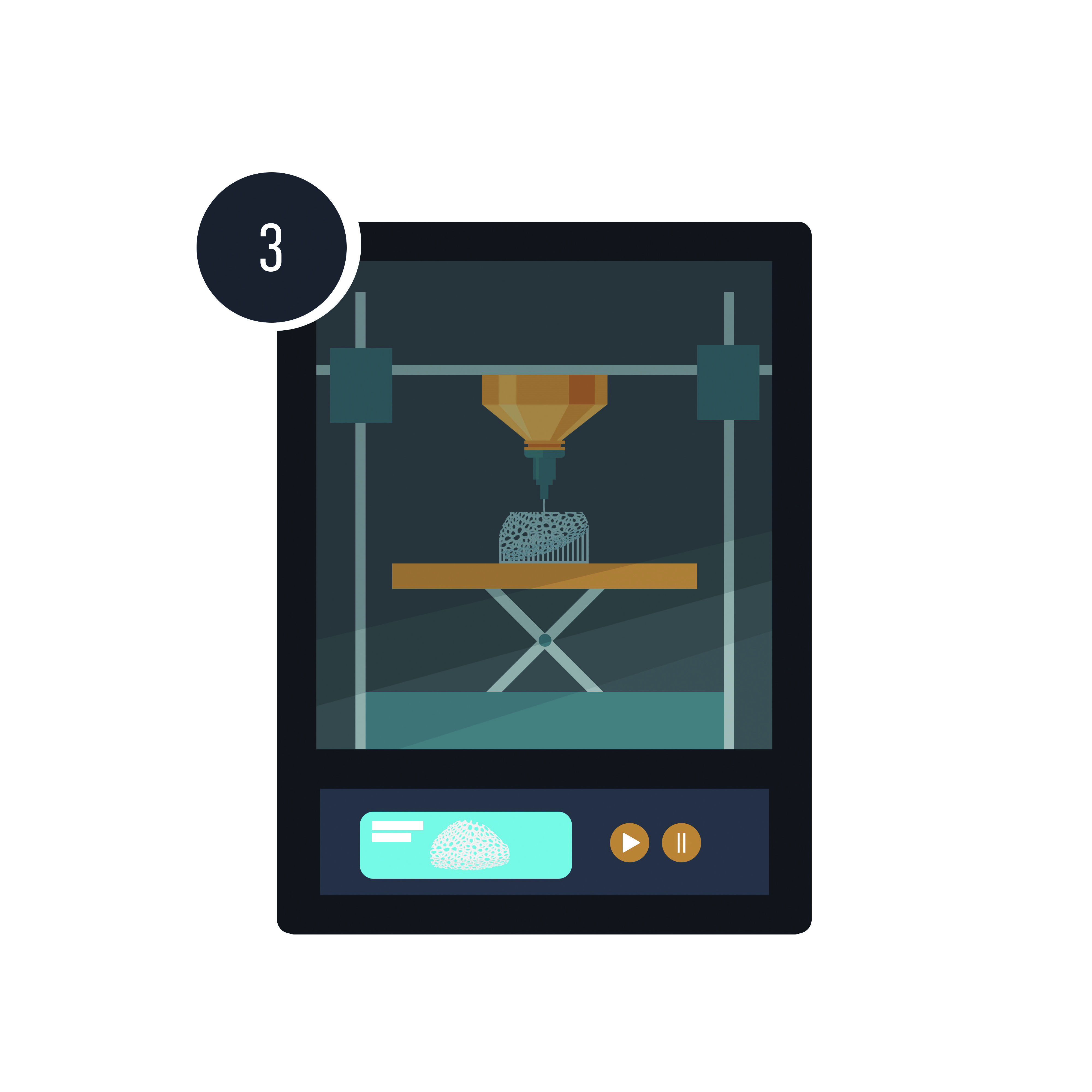
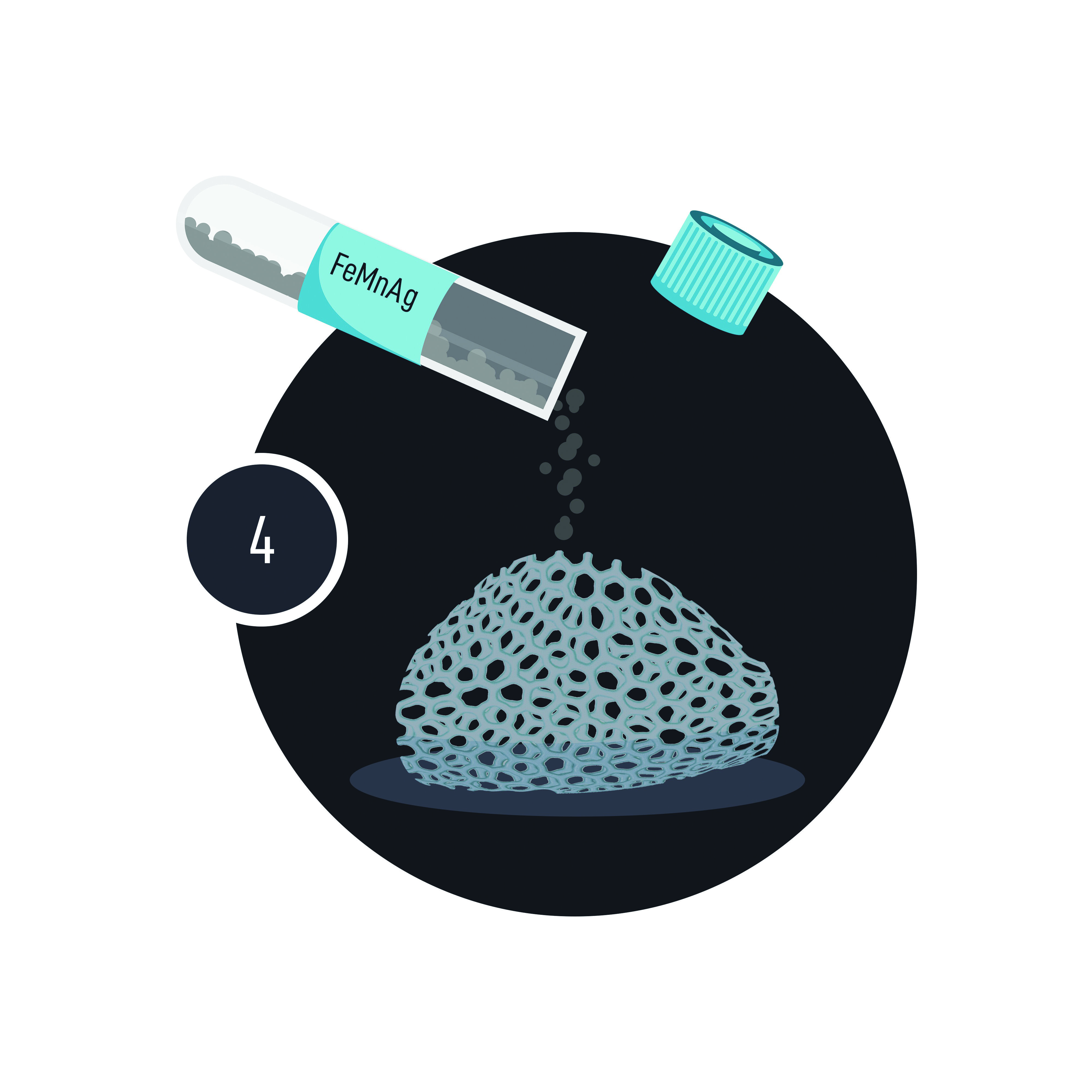
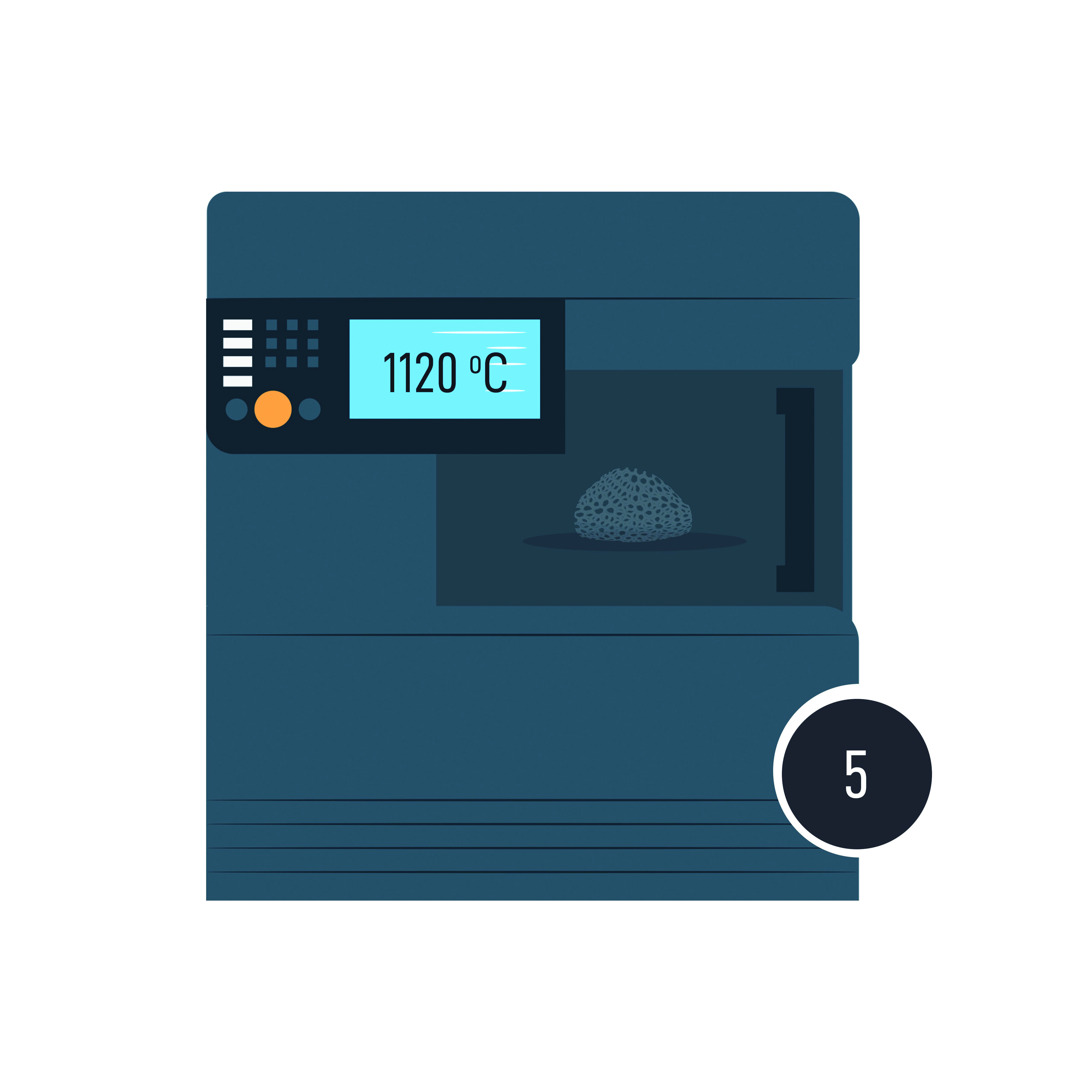
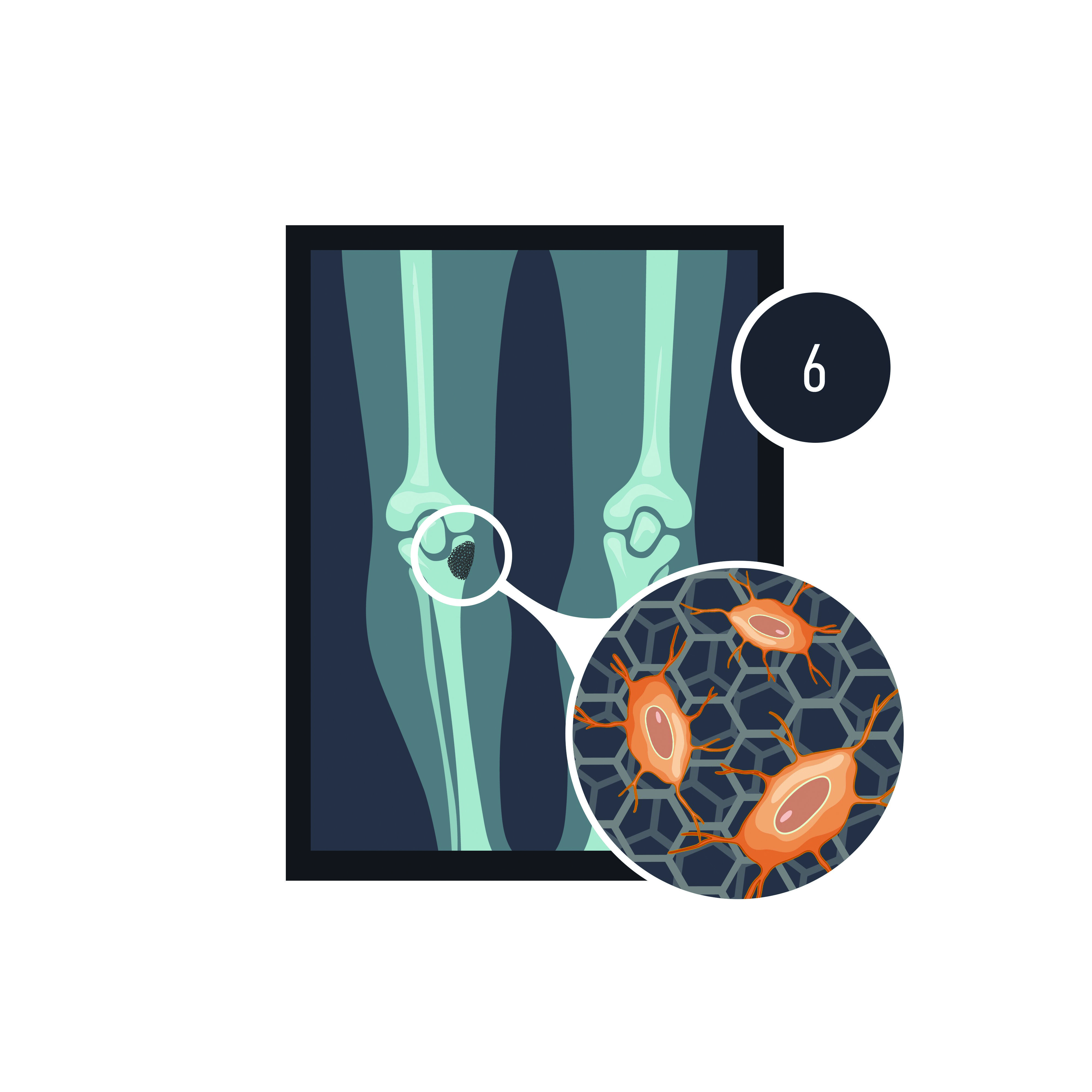
BioSA (R&I-2017-037T) is financed by the Malta Council for Science and Technology through the FUSION: R&I Technology Development Programme.
Further Reading
Wang, W., & Yeung, K. (2017). Bone grafts and biomaterials substitutes for bone defect repair: A review. Bioactive Materials, 2(4), 224-247. https://doi.org/10.1016/j.bioactmat.2017.05.007
Contact:
Prof. Ing. Joseph Buhagiar
t: 2340 2439
e: joseph.p.buhagiar@um.edu.mt
Comments are closed for this article!