Microfluidic devices manipulate small volumes of fluids, which are mainly used in biomedical application scenarios such as clinical diagnosis, drug delivery, and point-of-care testing. Creating such devices is a costly and challenging endeavour. The UM’s Department of Industrial and Manufacturing is investigating cost-efficient ways to produce microfluidic devices faster and a lot easier.
Lego blocks are extremely popular among children and adults alike. We can’t help but sit amused for hours building and trying out new shapes. Lego’s versatility enables us to create almost anything, from simple structures to true feats of home engineering, like small-scale reproductions of the popular Star Wars Millennium Falcon. In such endeavours, not every block is a simple cuboid shape. Similarly, other real-life products are rarely made with simple shapes in mind. Developing particular products that require a specific shape is a challenge uniquely fit for additive manufacturing, otherwise known as 3D printing. In essence, 3D printing can be considered as a highly personalized way to develop new types of Lego blocks designed to tackle specific problems.
As technology advances, products around us are becoming smaller, whilst tending to integrate more functions. To satisfy their specific functional and technical requirements, single components in such devices often necessitate the use of complex 3D internal structures, like hollow cavities at very small scales.
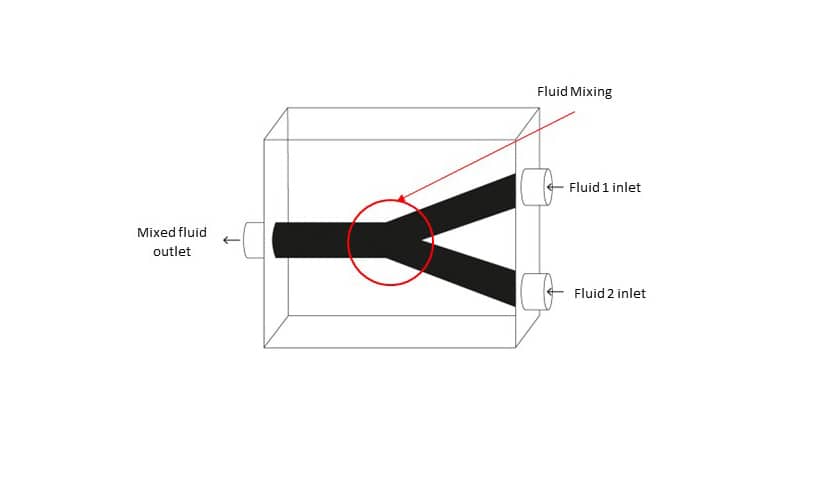
For example, microfluidic devices, similar to what is seen in the above image, are miniaturized systems that manipulate small volumes on fluids. One application is in point-of-care diagnostics. After collecting a small sample of blood, doctors introduce the blood sample to the microfluidic device. The device contains a network of tiny channels and chambers which the blood passes through. Within these channels, the blood sample might be mixed with reagents, antibodies, or specific markers to help doctors analyse how the blood reacts and develop a diagnosis. Creating such a product with intricate internal hollow features has been a difficult puzzle to crack in a cost-efficient manner. Fortunately, MICORE is around.
‘Our project is aimed at developing a cost-effective manufacturing process chain for components with hollow micro cavities’, explains Dr Ing. Pierre Vella, MICORE project coordinator and Senior Lecturer in the Faculty of Engineering, ‘We use 3D-printing along with injection moulding to manufacture these kinds of products in one step’. Along with Dr Ing. Pierre Vella, the project includes Key Expert, Prof. Arif Rochman (Associate Professor in the Faculty of Engineering) and Research Support Officer, Jeremy Farrugia. The research may have a vast number of applications, but their focus is primarily on the production of microfluidic devices, capable of delivering drugs, diagnosing, or even counting blood cells.
One Step Wonder
Micro-pumps could be found within microfluidic devices and are responsible for moving fluids through the microchannels. Microfluidic devices find their main application in biomedical applications. However, their manufacturing has serious drawbacks. ‘The production of micro-pumps for medical applications must be very precise,’ details Dr Ing. Vella, ‘Currently, the plastic pump with internal hollow functional features must first be split up into a number of different components/ layers so that we’ll be able to manufacture them separately using the injection moulding process. To have the whole pump, we must then assemble those layers together by welding, adhesive bonding, or mechanical fastening. There are a lot of steps. Therefore, it’s quite complex and relatively expensive.’ The MICORE team proposes a much easier yet elegant solution to this. In a way, it starts by manufacturing the product in reverse. Instead of manufacturing the solid parts of the object, Dr Ing. Vella and his colleagues start by 3D-printing the shape of the hollow spaces inside first using a soluble polymer, creating something called “the lost-core”. This ensures that the inner features of the component will be created during the injection moulding process. Next, the lost-core is placed inside the mould in predefined areas and subsequently a polymer material would be then injected over the lost-core. At the end of the process, the combined lost-core and polymeric material component are then submerged in a solvent where the core is dissolved, leaving polymer components with internal cavities in their place. This way, the process excludes the necessity for any additional (post-processing) steps such as welding or other assembly techniques since the device comes out in one piece. Therefore, it’s easier and faster to produce on a large scale. Prof. Rochman explains, ’Another advantage of this approach is that the shapes of the internal hollow features are directly replicated by the lost-core ensuring the precise flow rate of the fluid in the micro fluidic devices’.
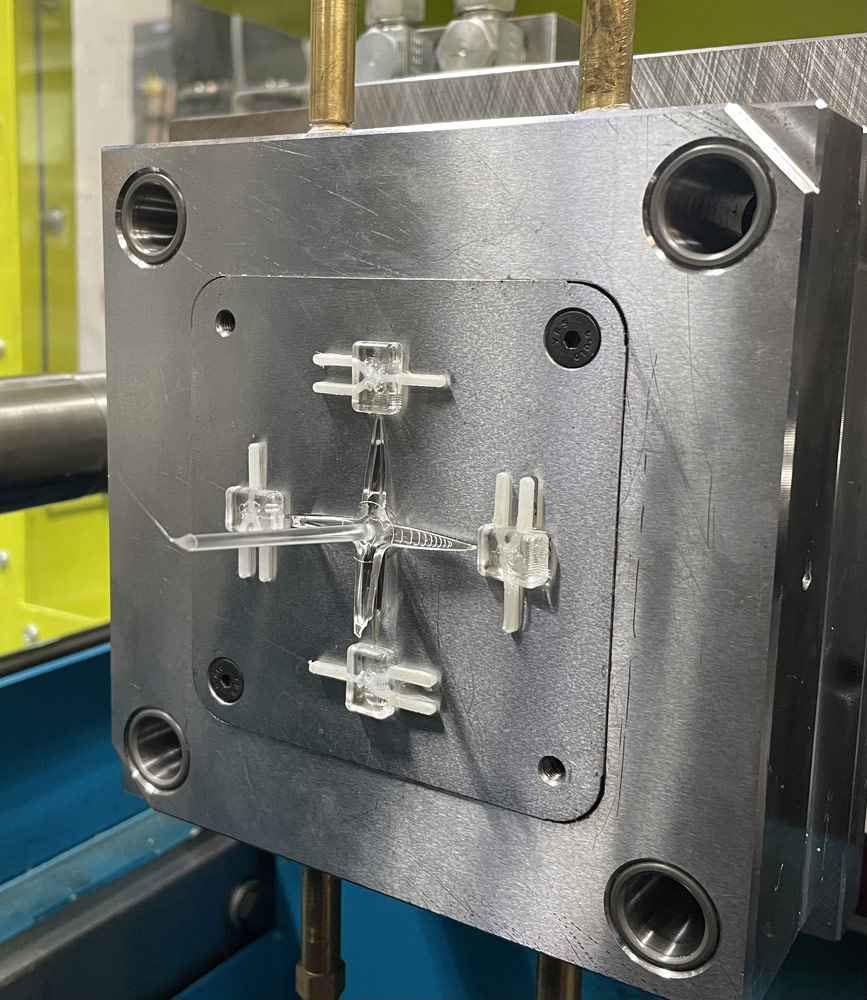
The Devil in the Details
After designing the basic procedure, researchers need to adjust techniques and materials to make sure these complex components are correctly printed, from both the inside and the outside. ‘The first challenge is printing the lost-core with fairly accurate dimensions because we are dealing with very small fluidic devices’, explains Prof. Rochman. A mistake can compromise the effectiveness of the biomedical microfluidic device.
The selection of the appropriate type of printer and core material is, consequently, of great relevance. The inner core is printed using a technology called Digital Light Processing (DLP), which works through a liquid resin that solidifies after being exposed to a light source. The chosen core material needs to be soluble so it can be dissolved later, leaving the required hollow cavity. The over-moulding of the core is done through the micro injection moulding process, where a polymeric material (such as transparent polymethyl methacrylate, ie. PMMA) is injected into the mould and encapsulates the lost-core.
There is also the question of other alternative candidate 3D printing processes and core materials which can be used in this process chain. The test core artefact used in this study is a Y-shaped channel, designed to mix two different fluids together. The team printed the test cores using both a filament-based material and a resin-based material to test different widths and print resolutions. ‘Strength and thermal resistance are other important factors to consider when choosing the different core materials since the material will be exposed to high injection pressure and temperatures during the injection moulding process’, Prof. Rochman explains further.
The success of this one-year-long project was demonstrating that the process chain is not only feasible but also advantageous when compared to alternative production methods. The results until now are only the tip of the iceberg! With the correct funding, the project can expand with more experimentation and technological development. Maybe one day, the production of these intricate components with complex macro and micro interior structures will be as easy as finding the correctly shaped Lego set! Until then, MICORE will keep on refining the building blocks of its process.
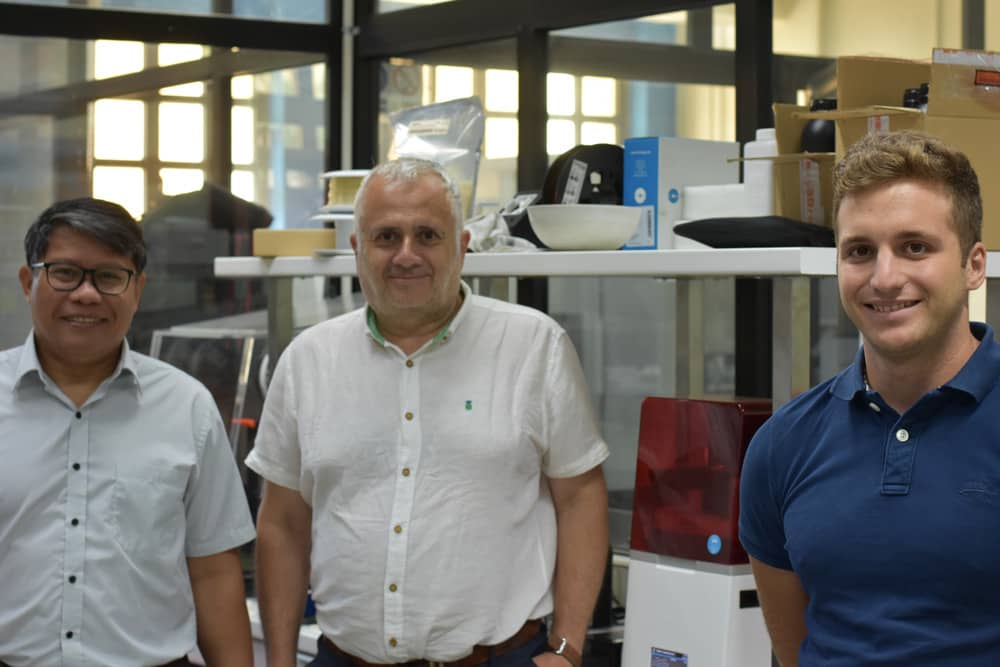
This project is funded by the Fusion R&I Research Excellence Programme of the Malta Council for Science and Technology (Project REP – 2022 – 011) and is led by the University of Malta Department of Industrial and Manufacturing Engineering.
Comments are closed for this article!