Unlocking the mysteries of the brain with MRI. Everything we think, say, or do depends on our brain. It is the most vital organ of our body but one of the least understood. Recent advances are changing things. With magnetic resonance imaging (MRI), scientists and researchers are getting an inside look into what makes us tick. Cassi Camilleri speaks to Dr Sonia Waiczies Chetcuti, Dr Helmar Waiczies and Prof. Kenneth Camilleri about their vision for experimental MRI in Malta. Illustrations by Sonya Hallett.
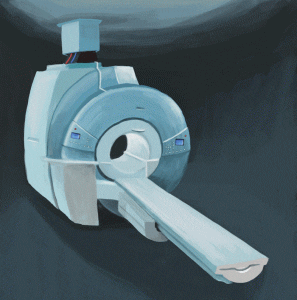
The brain is a unique machine. Its intricate wiring, made up of billions of neurons firing relentlessly, keeps it in constant contact with every part of the body. No supercomputer can compare. This level of complexity has made it deeply difficult for humans to study how the brain interacts with the body’s various systems. Treating it when something goes wrong is even more difficult.
This is where Dr Sonia Waiczies Chetcuti comes in. Starting her career in pharmacology, Sonia experimented with molecules found within cells with the long-term goal of developing new therapies for various ailments. However, the microscopic level at which she was working, as well as the uncertainty of whether or not her work would ultimately be used in the medical field, saw her interests wander: ‘I believed that looking at the organism as a whole brought you closer to the reality of life.’ It was within the field of neuro-immunology, the study of the interaction between the immune system and the nervous system, that she eventually found her niche.
‘What fascinated me is this balance in the immune system. On one hand, it can trigger a healthy response to protect the brain against harmful invading organisms, while on the other hand, it can go overboard, attacking itself.’ This is the principle of autoimmunity that underlies Sonia’s Ph.D. She believes that ‘understanding [this] will help us learn how to control it.’ For her Ph.D. (carried out at the Charité Medical University between 1999 and 2003) Sonia focused her attention on studying the autoimmune reaction in Multiple Sclerosis (MS) using animal models.
The pathology underlying MS sees the immune system trigger a chain reaction where white blood cells called T-cells are recruited by the Central Nervous System (CNS), consisting of the brain and spinal cord. The persistent presence of T-cells here leads to the damage of neurons, an insidious process which results in the disintegration of the fatty myelin sheath insulating the neuron—analogous to stripping a copper wire. Its removal inhibits the neuron from firing messages as quickly and efficiently as it normally would.
At this stage, lesions develop in the brain, and, when a sufficient number of neurons have been affected, clear symptoms begin to manifest, typically diplopia and ataxia, double vision and impaired bodily movement. The all important issue Sonia tackled in her research, and which is still unanswered, is: ‘What is this trigger that sets the immune system haywire? What is the root cause?’
While various scientists and researchers have looked into mapping molecular movement in cells when the illness hits, this highly focused approach has so far failed to properly shed light on the hows and the whys of MS. Many times, the work is disjointed, with professionals working separately, focusing on their own fields. Geneticists look at genetics. Clinicians and epidemiologists look at environmental and socioeconomic factors.
“Since MRI is completely non-invasive and makes no use of harmful ionising radiation, it allows researchers to take multiple scans and observe what is happening at various disease stages.”
But the reality is that ‘various factors need to be considered at the same time for an answer to be found,’ says Sonia. She likens their endeavour to that of looking for a needle in a haystack and, coincidently, the solution she applied elegantly fits both problems. In both cases, a very powerful magnet is required. Energised by the concept, Sonia sought to apply the use of MR technology to her study of MS.
Inspiration came in the shape of an article by Eric T. Ahrens in Nature Biotechnology called In vivo imaging platform for tracking immunotherapeutic cells. Using fluorine, an element not commonly found in nature, the researchers tagged dendritic cells and tracked their movements in a living mouse using MRI.
Sonia’s work on the animal model of MS had already succeeded in identifying a compound, extracellular-related kinase-1 (ERK-1), which has been shown to be essential to immune system regulation. Removing the molecule contributed to more severe autoimmune disease. This made certain immune cells more prone to attack self tissue in the body they reside in. A few years back, Sonia figured out that a principle would be beneficial to exploit in cancer, where the body replicates endlessly to unfortunate consequences: tumours.
It is in our interest that tumour tissue is destroyed by these immune cells. To this end, she studied this concept (at the Max Delbrück Center for Molecular Medicine), whereby mice with gliomas (a type of brain tumour) were given these immune cells which had ERK-1 removed as a cellular therapy. This resulted in a halt to the tumour’s growth. Now they are using fluorine to track these potent cell therapies in animal models by using various MRI techniques. In the case of MS, neuronal damage can be measured by quantifying the performance of specific regions of the brain with the use of functional MRI. Magnetic resonance spectroscopy also does this by looking at variations in the metabolites found in the brain. Since MRI is completely non-invasive and makes no use of harmful ionising radiation, it allows researchers to take multiple scans and observe what is happening at various disease stages. This is not just in the case of MS; a plethora of other diseases can be studied in this manner, including Alzheimer’s and Parkinson’s disease.
This leaves little doubt as to the massive potential MRI technology holds. Used as an experimental tool, it enables researchers to develop treatments. As a diagnostic tool, it allows doctors to make better diagnoses and ultimately provide better care.
Having said that, this use of experimental MRI technology is not streamlined. Sonia is quick to point out that a so-called ‘clinico-radiological paradox’ remains, meaning that results from the still-evolving MRI techniques have not yet fallen in line with clinical knowledge. In some cases, a patient would come in with obvious symptoms of MS but no lesions would be indicated on the MRI. On the other hand, someone barely showing any symptoms could produce an MRI which shows a brain full of lesions.
On a more technical level, one of the biggest hurdles with MRI are the limitations in the laws of physics that govern it. This is where the need for a different set of skills becomes clear. In Sonia’s case those skills were to be found very close to home. Her husband, Dr Helmar Waiczies, was already working in the field of MRI as a Radio-frequency engineer. His shared interest in immune systems made him an invaluable source of information.
MRI Physics
MRI is a non-invasive medical test that aids doctors and physicians in their diagnoses and treatment of various medical conditions. During an MRI scan, the subject lies in a strong magnetic field with radio-frequency waves directed at them. The magnets in the MRI scanner line up the protons (H+ ions) in the body in the same direction because they are sensitive to a magnetic field.
Short bursts of radio waves are then sent to particular areas of the body, knocking the protons out of alignment. When the radio waves are turned off, the protons then realign and in so doing they send out radio signals, which are picked up by receiver coils.
These signals provide information about the exact location of the protons in the body. Then, in the same way that millions of pixels on a computer screen can create complex pictures, the signals from the millions of protons in the body are combined to create a detailed image. These images enable physicians to evaluate various parts of the body and determine the presence or development of certain diseases.
A problem they needed to overcome was that of signal-to-noise ratio, a standard limitation in MRI, says Helmar. A bad signal-to-noise ratio means that the MRI cannot pick up signals from the body due to the high level of noise being emitted by the electronics, the cables and other components in the machine. This makes a diagnosis nearly impossible; the image would be unclear. ‘The noise is like the lawn in a garden. If it is too high, flowers, signals, will no longer be seen.’ To deal with the issue, Sonia and Helmar developed a radio frequency coil which they dubbed the ‘Shingled leg coil.’ Its design eliminated the need for extra electrical components on the probe structure. This reduced noise, thus allowing for signals to be picked up better, and resulting in sharper images.
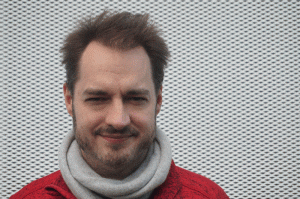
‘The current technological restrictions of MRI means that the role of physicists in this work cannot be understated,’ says Sonia. Engineers need medical professionals to know what ailments can be improved using technology. Biologists need to know how far technology can go. In MRI research this interdisciplinary approach is crucial. This is perfectly in line with what Prof. Kenneth Camilleri, from the Centre for Biomedical Cybernetics at the University of Malta, thinks. The Centre brings together engineers and clinicians to open the channels of communication and collaboration. It promotes the sharing of ideas, fosters discussions, and pushes for research to be applied in the real world. Camilleri is working with Sonia in bringing her vision for the development of MRI technology to Malta. Through the Magnetic Resonance Imaging Group Initiative, they are working together to establish an experimental MRI Centre at the University of Malta.
The challenge is difficult because it needs around €3–5 million to set up. There would also be recurring maintenance costs of half a million Euro each year. ‘There is no denying that finance has been a problem. However, the returns from having such a facility on the Island would come in many forms,’ points out Camilleri.
“Camilleri is working with Sonia in bringing her vision for the development of MRI technology to Malta.”
Not only would the Centre put Malta on the map in the field of medical and engineering research but it would also provide various services both locally and internationally. Clinical drug studies would become a possibility, attracting pharmaceutical companies from all around the world. This would offer a new opportunity for the provision of service to various investors.
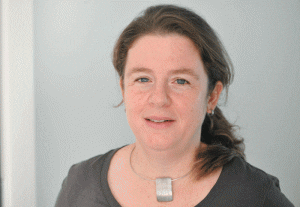
The Centre would also benefit cultural heritage agencies such as Heritage Malta. Artefacts can be scanned in 3D to help restoration and conservation effects, thereby indirectly providing a service to the local tourism industry.
According to Sonia, however, one of the most important applications of the centre will remain in healthcare. ‘We are living in an aging society. So we really have to invest more in screening people.’ In Germany, regular screenings help identify disease before it becomes incurable. This regular testing also provides a wealth of data for researchers to develop a better understanding of various diseases. In Malta, this has not been adopted systematically. However, with the country’s reputation as a retirement haven becoming entrenched, this makes the centre even more important.
There is no denying that care is crucial. However, being able to differentiate between diseases better and earlier will prove essential in the long-run. It is about working backwards and trying to improve on what is already known to develop better treatments and improve people lives. As Sonia rightly says, ‘It’s about taking the bull by the horns.’
Auto-immunity: How does it happen?
Our immune system is there to resist attack from invading microorganisms such as viruses, bacteria, and parasites. White blood cells, particularly T-lymphocytes, are called upon by dendritic cells, which tag the invaders to make it clear what the lymphocytes should attack. Dendritic cells will ‘present’ uniquely identifiable proteins, antigens, found on the invading organisms, much like a cellular ID card. The immune system is then able to make a very important distinction between what is self and what is non-self, based on these antigens. This is called tolerance, whereby self is tolerated, and non-self, is attacked. It is the only discriminatory act the immune system makes.
Autoimmunity is what happens when those self antigens, are, for some as yet incompletely understood reason, now seen as non-self, and so, would be targeted as if they were just another troublesome bug. The cellular process that leads to autoimmunity diseases can be normal in a healthy body.
For example, autophagy (the planned and controlled death of cells and their clearing) sees potentially harmful products of cell breakdown cleared out. In these cases, even though it is our own cells that are being done away with, they are cells which are no longer useful or needed, and so tolerance is still maintained. The problem with autoimmune disease is when no distinction is made anymore between redundant cells and fully functional cells. The functional cells then start being cleared out for no good reason.
Why this happens is still largely unknown, but contributing factors include inheritance of genes that could disrupt different tolerance pathways (genetic predisposition), and some environmental trigger. Apart from this autophagy process others have been proposed to explain why the immune system can go berserk leading to autoimmune disease.
Further reading
- Bushong, S. and Clarke, G. (2014). Magnetic resonance imaging.
St. Louis: Elsevier Mosby. - Waiczies, S. and Waiczies, H. (2011). Understanding the Pathogenesis of Neuroinflammation using Magnetic Resonance Imaging. Malta Medical Journal 23, 3:56-60.
- Ahrens, E., Flores, R., Xu, H. and Morel, P. (2005). In vivo imaging platform for tracking immunotherapeutic cells. Nature Biotechnology, 23(8), pp.983-987.
Comments are closed for this article!